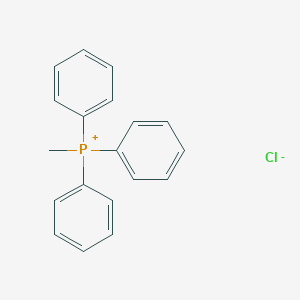
Methyltriphenylphosphonium chloride
概要
説明
Methyltriphenylphosphonium chloride is an organophosphorus compound with the chemical formula CH₃P(C₆H₅)₃Cl. It is a white crystalline solid that is soluble in methanol. This compound is widely used in synthetic chemistry as a phase transfer catalyst and in various other applications due to its unique chemical properties .
作用機序
Target of Action
Methyltriphenylphosphonium chloride (MTPC) is a versatile compound with several targets. It is primarily used as a phase transfer catalyst in synthetic chemistry . It also acts as a corrosion inhibitor for iron . Furthermore, it has been reported to have an antimitochondrial effect , suggesting a potential role in neoplasm inhibition .
Mode of Action
MTPC’s mode of action depends on its application. As a phase transfer catalyst, it facilitates the migration of a reactant from one phase into another where reaction occurs . When used for corrosion inhibition, it likely forms a protective layer on the iron surface, preventing corrosive substances from reaching the metal .
Biochemical Pathways
Its role as a reactant for intramolecular nitrone cycloaddition suggests it may be involved in various organic synthesis pathways . Its antimitochondrial effect implies it may interfere with energy production pathways within the cell .
Pharmacokinetics
Like many phase transfer catalysts, its effectiveness would depend on its ability to distribute between different phases and facilitate reactant transfer . Its bioavailability would likely be influenced by factors such as solubility, absorption, distribution, metabolism, and excretion.
Result of Action
The result of MTPC’s action is context-dependent. In synthetic chemistry, it enables reactions that might otherwise be slow or impossible . In corrosion inhibition, it protects iron surfaces from damage . Its antimitochondrial effect could potentially lead to cell death, providing a basis for its potential use in cancer therapy .
Action Environment
The action of MTPC can be influenced by various environmental factors. For instance, the efficiency of phase transfer catalysis can be affected by the nature of the solvent, temperature, and the presence of other substances . Its corrosion inhibition effect might be influenced by the corrosiveness of the environment . The effectiveness of its antimitochondrial action could be modulated by factors within the cellular environment .
生化学分析
Biochemical Properties
Methyltriphenylphosphonium chloride interacts with various biomolecules in biochemical reactions. For instance, it has been found to inhibit the Krebs cycle enzyme 2-oxoglutarate dehydrogenase complex (OGDHC) with an estimated IC50 of 3.93 [3.70–4.17] mM . This interaction affects the metabolic processes within cells, influencing the production of energy and other essential biochemical reactions .
Cellular Effects
This compound has significant effects on cellular functions. It has been observed to cause a progressive inhibition of cellular respiration in adherent cells without a marked effect on mitochondrial coupling . This impact on cellular respiration can influence various cellular processes, including cell signaling pathways, gene expression, and cellular metabolism .
Molecular Mechanism
The molecular mechanism of action of this compound involves its interaction with enzymes and other biomolecules at the molecular level. For instance, it inhibits the activity of the OGDHC enzyme, which plays a crucial role in the Krebs cycle . This inhibition can lead to changes in cellular metabolism and energy production .
Temporal Effects in Laboratory Settings
The effects of this compound can change over time in laboratory settings. For instance, micromolar concentrations of this compound cause a progressive inhibition of cellular respiration
Metabolic Pathways
This compound is involved in the Krebs cycle, a crucial metabolic pathway in cells . It interacts with the OGDHC enzyme, affecting the flux of this metabolic pathway
準備方法
Methyltriphenylphosphonium chloride is typically synthesized by reacting triphenylphosphine with methyl chloride. The reaction is carried out under normal conditions and yields a high product. The general reaction is as follows:
P(C6H5)3+CH3Cl→CH3P(C6H5)3Cl
In industrial settings, the production of this compound involves similar synthetic routes but on a larger scale, ensuring high purity and yield .
化学反応の分析
Methyltriphenylphosphonium chloride undergoes various types of chemical reactions, including:
Oxidation: It can be oxidized to form phosphine oxides.
Reduction: It can be reduced to form phosphines.
Substitution: It participates in nucleophilic substitution reactions, where the chloride ion can be replaced by other nucleophiles.
Common reagents and conditions used in these reactions include strong oxidizing agents for oxidation, reducing agents for reduction, and various nucleophiles for substitution reactions. The major products formed from these reactions depend on the specific reagents and conditions used .
科学的研究の応用
Methyltriphenylphosphonium chloride has a wide range of scientific research applications:
類似化合物との比較
Methyltriphenylphosphonium chloride can be compared with other similar compounds such as:
Methyltriphenylphosphonium bromide: Similar in structure but contains a bromide ion instead of a chloride ion.
Methyltriphenylphosphonium iodide: Contains an iodide ion instead of a chloride ion.
Ethyltriphenylphosphonium bromide: Contains an ethyl group instead of a methyl group and a bromide ion instead of a chloride ion.
The uniqueness of this compound lies in its specific chemical properties and applications, particularly its use as a phase transfer catalyst and its antimitochondrial effect .
特性
IUPAC Name |
methyl(triphenyl)phosphanium;chloride | |
---|---|---|
Source | PubChem | |
URL | https://pubchem.ncbi.nlm.nih.gov | |
Description | Data deposited in or computed by PubChem | |
InChI |
InChI=1S/C19H18P.ClH/c1-20(17-11-5-2-6-12-17,18-13-7-3-8-14-18)19-15-9-4-10-16-19;/h2-16H,1H3;1H/q+1;/p-1 | |
Source | PubChem | |
URL | https://pubchem.ncbi.nlm.nih.gov | |
Description | Data deposited in or computed by PubChem | |
InChI Key |
QRPRIOOKPZSVFN-UHFFFAOYSA-M | |
Source | PubChem | |
URL | https://pubchem.ncbi.nlm.nih.gov | |
Description | Data deposited in or computed by PubChem | |
Canonical SMILES |
C[P+](C1=CC=CC=C1)(C2=CC=CC=C2)C3=CC=CC=C3.[Cl-] | |
Source | PubChem | |
URL | https://pubchem.ncbi.nlm.nih.gov | |
Description | Data deposited in or computed by PubChem | |
Molecular Formula |
C19H18ClP | |
Source | PubChem | |
URL | https://pubchem.ncbi.nlm.nih.gov | |
Description | Data deposited in or computed by PubChem | |
DSSTOX Substance ID |
DTXSID70432258 | |
Record name | Methyltriphenylphosphonium chloride | |
Source | EPA DSSTox | |
URL | https://comptox.epa.gov/dashboard/DTXSID70432258 | |
Description | DSSTox provides a high quality public chemistry resource for supporting improved predictive toxicology. | |
Molecular Weight |
312.8 g/mol | |
Source | PubChem | |
URL | https://pubchem.ncbi.nlm.nih.gov | |
Description | Data deposited in or computed by PubChem | |
CAS No. |
1031-15-8 | |
Record name | Methyltriphenylphosphonium chloride | |
Source | CAS Common Chemistry | |
URL | https://commonchemistry.cas.org/detail?cas_rn=1031-15-8 | |
Description | CAS Common Chemistry is an open community resource for accessing chemical information. Nearly 500,000 chemical substances from CAS REGISTRY cover areas of community interest, including common and frequently regulated chemicals, and those relevant to high school and undergraduate chemistry classes. This chemical information, curated by our expert scientists, is provided in alignment with our mission as a division of the American Chemical Society. | |
Explanation | The data from CAS Common Chemistry is provided under a CC-BY-NC 4.0 license, unless otherwise stated. | |
Record name | Methyltriphenylphosphonium chloride | |
Source | ChemIDplus | |
URL | https://pubchem.ncbi.nlm.nih.gov/substance/?source=chemidplus&sourceid=0001031158 | |
Description | ChemIDplus is a free, web search system that provides access to the structure and nomenclature authority files used for the identification of chemical substances cited in National Library of Medicine (NLM) databases, including the TOXNET system. | |
Record name | Methyltriphenylphosphonium chloride | |
Source | EPA DSSTox | |
URL | https://comptox.epa.gov/dashboard/DTXSID70432258 | |
Description | DSSTox provides a high quality public chemistry resource for supporting improved predictive toxicology. | |
Record name | Methyltriphenylphosphonium chloride | |
Source | European Chemicals Agency (ECHA) | |
URL | https://echa.europa.eu/substance-information/-/substanceinfo/100.101.722 | |
Description | The European Chemicals Agency (ECHA) is an agency of the European Union which is the driving force among regulatory authorities in implementing the EU's groundbreaking chemicals legislation for the benefit of human health and the environment as well as for innovation and competitiveness. | |
Explanation | Use of the information, documents and data from the ECHA website is subject to the terms and conditions of this Legal Notice, and subject to other binding limitations provided for under applicable law, the information, documents and data made available on the ECHA website may be reproduced, distributed and/or used, totally or in part, for non-commercial purposes provided that ECHA is acknowledged as the source: "Source: European Chemicals Agency, http://echa.europa.eu/". Such acknowledgement must be included in each copy of the material. ECHA permits and encourages organisations and individuals to create links to the ECHA website under the following cumulative conditions: Links can only be made to webpages that provide a link to the Legal Notice page. | |
Record name | Methyltriphenylphosphonium chloride | |
Source | European Chemicals Agency (ECHA) | |
URL | https://echa.europa.eu/information-on-chemicals | |
Description | The European Chemicals Agency (ECHA) is an agency of the European Union which is the driving force among regulatory authorities in implementing the EU's groundbreaking chemicals legislation for the benefit of human health and the environment as well as for innovation and competitiveness. | |
Explanation | Use of the information, documents and data from the ECHA website is subject to the terms and conditions of this Legal Notice, and subject to other binding limitations provided for under applicable law, the information, documents and data made available on the ECHA website may be reproduced, distributed and/or used, totally or in part, for non-commercial purposes provided that ECHA is acknowledged as the source: "Source: European Chemicals Agency, http://echa.europa.eu/". Such acknowledgement must be included in each copy of the material. ECHA permits and encourages organisations and individuals to create links to the ECHA website under the following cumulative conditions: Links can only be made to webpages that provide a link to the Legal Notice page. | |
Record name | METHYLTRIPHENYLPHOSPHONIUM CHLORIDE | |
Source | FDA Global Substance Registration System (GSRS) | |
URL | https://gsrs.ncats.nih.gov/ginas/app/beta/substances/0Z16KIM767 | |
Description | The FDA Global Substance Registration System (GSRS) enables the efficient and accurate exchange of information on what substances are in regulated products. Instead of relying on names, which vary across regulatory domains, countries, and regions, the GSRS knowledge base makes it possible for substances to be defined by standardized, scientific descriptions. | |
Explanation | Unless otherwise noted, the contents of the FDA website (www.fda.gov), both text and graphics, are not copyrighted. They are in the public domain and may be republished, reprinted and otherwise used freely by anyone without the need to obtain permission from FDA. Credit to the U.S. Food and Drug Administration as the source is appreciated but not required. | |
Retrosynthesis Analysis
AI-Powered Synthesis Planning: Our tool employs the Template_relevance Pistachio, Template_relevance Bkms_metabolic, Template_relevance Pistachio_ringbreaker, Template_relevance Reaxys, Template_relevance Reaxys_biocatalysis model, leveraging a vast database of chemical reactions to predict feasible synthetic routes.
One-Step Synthesis Focus: Specifically designed for one-step synthesis, it provides concise and direct routes for your target compounds, streamlining the synthesis process.
Accurate Predictions: Utilizing the extensive PISTACHIO, BKMS_METABOLIC, PISTACHIO_RINGBREAKER, REAXYS, REAXYS_BIOCATALYSIS database, our tool offers high-accuracy predictions, reflecting the latest in chemical research and data.
Strategy Settings
Precursor scoring | Relevance Heuristic |
---|---|
Min. plausibility | 0.01 |
Model | Template_relevance |
Template Set | Pistachio/Bkms_metabolic/Pistachio_ringbreaker/Reaxys/Reaxys_biocatalysis |
Top-N result to add to graph | 6 |
Feasible Synthetic Routes
Q1: What is the primary use of Methyltriphenylphosphonium chloride in organic synthesis?
A1: this compound serves as a precursor to the reactive Wittig reagent, methylenetriphenylphosphorane. This ylide plays a crucial role in organic synthesis for olefination reactions, specifically converting carbonyl compounds (aldehydes and ketones) into alkenes. [, ]
Q2: Can you provide examples of specific reactions where this compound plays a key role?
A2: Certainly! One example is the reaction of this compound with isocyanide dichlorides. This reaction leads to the formation of N-substituted (triphenylphosphoranylidene)ketenimines, highlighting the versatility of this compound in synthesizing structurally diverse molecules. [] Additionally, it reacts with nitrosyl chloride to produce α-substituted α-hydroxyiminothis compound. []
Q3: How does the structure of this compound relate to its reactivity?
A3: The key structural feature contributing to its reactivity is the presence of the phosphonium cation (PPh3Me)+. This cation, when treated with a strong base, readily generates the corresponding ylide, methylenetriphenylphosphorane, due to the relatively acidic protons on the methyl group adjacent to the positively charged phosphorus atom.
Q4: Has the electron spin resonance (ESR) spectrum of the methyltriphenylphosphonium radical cation been studied?
A4: Yes, studies have investigated the ESR spectrum of the methyltriphenylphosphonium radical cation, generated by X-irradiation of a single crystal of the compound. These investigations revealed insights into the radical's structure and dynamics, particularly the reorientation of the methylene group. []
Q5: Are there any analytical techniques mentioned in the research that are specifically used to study this compound and its derivatives?
A5: The research highlights the use of several analytical techniques, including:
- Nuclear Magnetic Resonance (NMR) Spectroscopy: This technique, particularly 13C-NMR, helps determine the structure of compounds like N-substituted (triphenylphosphoranylidene)ketenimines derived from this compound. Coupling constants obtained from the spectra provide valuable information about the connectivity and electronic environment of the atoms within the molecule. []
- Atomic Absorption Spectrophotometry (AAS): This technique is utilized for the quantitative determination of trace amounts of metals, such as iridium and ruthenium, in rhodium sponge samples. This compound is employed as an extractant in this process. []
- X-ray Crystallography: This technique provides detailed structural information about this compound and its derivatives in their solid state. It allows researchers to determine bond lengths, bond angles, and overall molecular geometry. [, ]
Q6: Are there any applications of this compound in material science?
A6: While not directly addressed in the provided research, derivatives of this compound have shown potential in material science. For instance, compounds containing this moiety have been explored for their complexation abilities with metals like palladium and platinum on silica gel, indicating their potential use in heterogeneous catalysis or sensor development. []
Q7: Has this compound been explored for its use in deep eutectic solvents (DES)?
A7: While the provided abstracts don't mention specific applications in DES systems, one study touches upon the use of DES for glycerol extraction from biodiesel. [] This suggests a potential area of exploration for this compound-based ionic liquids or DES systems, particularly for their application in sustainable chemistry and green solvents. Further research would be needed to assess their suitability and efficacy in such applications.
試験管内研究製品の免責事項と情報
BenchChemで提示されるすべての記事および製品情報は、情報提供を目的としています。BenchChemで購入可能な製品は、生体外研究のために特別に設計されています。生体外研究は、ラテン語の "in glass" に由来し、生物体の外で行われる実験を指します。これらの製品は医薬品または薬として分類されておらず、FDAから任何の医療状態、病気、または疾患の予防、治療、または治癒のために承認されていません。これらの製品を人間または動物に体内に導入する形態は、法律により厳格に禁止されています。これらのガイドラインに従うことは、研究と実験において法的および倫理的な基準の遵守を確実にするために重要です。