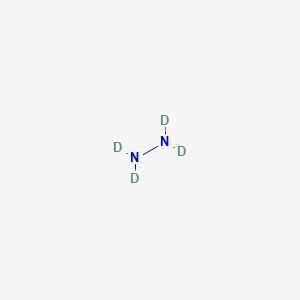
1,1,2,2-Tetradeuteriohydrazine
カタログ番号 B089299
CAS番号:
13762-95-3
分子量: 36.07 g/mol
InChIキー: OAKJQQAXSVQMHS-JBISRTOLSA-N
注意: 研究専用です。人間または獣医用ではありません。
概要
説明
Molecular Structure Analysis
The molecular formula of 1,1,2,2-Tetradeuteriohydrazine is H4N2. It has a molecular weight of 36.07 g/mol.Safety and Hazards
特性
IUPAC Name |
1,1,2,2-tetradeuteriohydrazine | |
---|---|---|
Source | PubChem | |
URL | https://pubchem.ncbi.nlm.nih.gov | |
Description | Data deposited in or computed by PubChem | |
InChI |
InChI=1S/H4N2/c1-2/h1-2H2/i/hD4 | |
Source | PubChem | |
URL | https://pubchem.ncbi.nlm.nih.gov | |
Description | Data deposited in or computed by PubChem | |
InChI Key |
OAKJQQAXSVQMHS-JBISRTOLSA-N | |
Source | PubChem | |
URL | https://pubchem.ncbi.nlm.nih.gov | |
Description | Data deposited in or computed by PubChem | |
Canonical SMILES |
NN | |
Source | PubChem | |
URL | https://pubchem.ncbi.nlm.nih.gov | |
Description | Data deposited in or computed by PubChem | |
Isomeric SMILES |
[2H]N([2H])N([2H])[2H] | |
Source | PubChem | |
URL | https://pubchem.ncbi.nlm.nih.gov | |
Description | Data deposited in or computed by PubChem | |
Molecular Formula |
H4N2 | |
Source | PubChem | |
URL | https://pubchem.ncbi.nlm.nih.gov | |
Description | Data deposited in or computed by PubChem | |
Molecular Weight |
36.070 g/mol | |
Source | PubChem | |
URL | https://pubchem.ncbi.nlm.nih.gov | |
Description | Data deposited in or computed by PubChem | |
Product Name |
Hydrazine-D4 |
Synthesis routes and methods I
Procedure details
The starting material, 5,5-diphenyl-2-imidazolin-2-ylhydrazine hydrobromide, is prepared as follows: 4,4-diphenylimidazolin-2-thione, prepared by the method of Marshall, J. Am. Chem. Soc. 78 3696 (1956), is treated with ethyl bromide in refluxing ethanol to yield 5,5-diphenyl-2-ethylthio-2-imidazoline hydrobromide, which upon treatment with hydrazine in refluxing ethanol gives the desired intermediate, melting point 227°-⟦8°⟧228° C.
Name
5,5-diphenyl-2-imidazolin-2-ylhydrazine hydrobromide
Quantity
0 (± 1) mol
Type
reactant
Reaction Step One
Name
Synthesis routes and methods II
Procedure details
The starting material, 4-methyl-2-imidazolin-2-ylhydrazine hydrochloride, is prepared as follows: 4-methylimidazolidin-2-thione and methyl iodide react in refluxing ethanol to give 4-methyl-2-methylthio-2-imidazoline hydroiodide, which upon treatment with hydrazine in boiling ethanol yields 4-methyl-2-imidazolin-2-ylhydrazine hydroiodide. Subsequent treatment with silver chloride in aqueous or ethanol solution then gives the hydrochloride salt melting at 128°-130° C.
Name
4-methyl-2-imidazolin-2-ylhydrazine hydrochloride
Quantity
0 (± 1) mol
Type
reactant
Reaction Step One
Name
Synthesis routes and methods III
Procedure details
The preparation of the starting material, 1,4,5,6-tetrahydropyrimidin-2-ylhydrazine hydrochloride, is carried out as follows: 1,4,5,6-tetrahydropyrimidin-2-thione and methyl chloride (excess) are added to isopropanol and the mixture heated in an autoclave at 170°-180° C. for hours. Cooling to 4° C. yields a precipitate of 2-methylthio-1,4,5,6-tetrahydropyrimidine hydrochloride, which upon treatment with hydrazine in boiling ethanol yields the desired intermediate melting at 191°-192° C.
Name
1,4,5,6-tetrahydropyrimidin-2-ylhydrazine hydrochloride
Quantity
0 (± 1) mol
Type
reactant
Reaction Step One
Name
Synthesis routes and methods IV
Procedure details
The starting material, 4-phenyl-2-imidazolin-2-ylhydrazine hydroiodide, is prepared as follows: 4-phenylimidazolidine-2-thione and methyliodide in refluxing ethanol yield 2-methylthio-4-phenyl-2-imidazolin hydroiodide, which upon treatment with hydrazine in boiling ethanol yields the desired intermediate, melting at 107°-108° C.
Name
4-phenyl-2-imidazolin-2-ylhydrazine hydroiodide
Quantity
0 (± 1) mol
Type
reactant
Reaction Step One
Name
Synthesis routes and methods V
Procedure details
70 ml of purified water was added to 8 g of anhydrous copper acetate (manufactured by Wako Pure Chemical Industries, Ltd.). Thereto was added 2 ml of 64 wt % hydrazine hydrate while stirring at 60° C. so as to give a molar ratio of hydrazine to copper acetate of 0.9, thereby carrying out the reaction to obtain a precipitate of cuprous oxide. The average primary particle diameter and the average secondary particle diameter were 50 nm and 180 μm, respectively. The average secondary particle diameter in the colloidal dispersion, obtained in the same manner as in Example 1, was 95 nm.
試験管内研究製品の免責事項と情報
BenchChemで提示されるすべての記事および製品情報は、情報提供を目的としています。BenchChemで購入可能な製品は、生体外研究のために特別に設計されています。生体外研究は、ラテン語の "in glass" に由来し、生物体の外で行われる実験を指します。これらの製品は医薬品または薬として分類されておらず、FDAから任何の医療状態、病気、または疾患の予防、治療、または治癒のために承認されていません。これらの製品を人間または動物に体内に導入する形態は、法律により厳格に禁止されています。これらのガイドラインに従うことは、研究と実験において法的および倫理的な基準の遵守を確実にするために重要です。