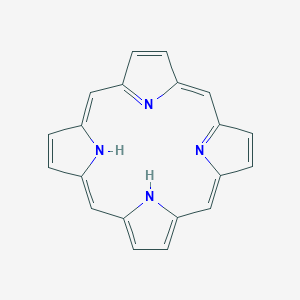
ポルフィン
概要
説明
Porphine, also known as porphin, is an organic compound with the empirical formula C20H14N4. It is a heterocyclic and aromatic molecule, consisting of a flat macrocycle formed by four pyrrole-like rings joined by four methine bridges. This structure makes porphine the simplest of the tetrapyrroles . Porphine is the parent compound of the porphyrin family, which includes many biologically significant molecules such as heme and chlorophyll .
科学的研究の応用
Porphine and its derivatives have a wide range of applications in scientific research:
作用機序
Target of Action
Porphine, also known as 21H,23H-Porphine, is a simple and non-substituted form of porphyrin . Porphyrins are a group of heterocyclic macrocycle organic compounds that play crucial roles in living organisms . The primary targets of porphine are the various enzymes and proteins where it acts as a prosthetic group . For instance, in hemoproteins, heme, a derivative of porphine, carries oxygen in the bloodstream .
Mode of Action
Porphine and its derivatives interact with their targets by fitting into the protein structures and participating in their functions . For example, in hemoglobin, the iron atom in the heme group (a derivative of porphine) binds to oxygen molecules and helps in their transport .
Biochemical Pathways
Porphine is involved in several biochemical pathways. It plays a pivotal role in the generation, storage, and use of oxygen, which is essential to life . In plants, chlorophyll, a derivative of porphine, is involved in light harvesting and electron transfer in photosynthesis .
Pharmacokinetics
Porphyrins, in general, are known to have a wide distribution in the body due to their involvement in various biological functions .
Result of Action
The action of porphine results in several molecular and cellular effects. Its role as a component of heme and chlorophyll leads to its involvement in vital processes such as oxygen transport and photosynthesis . These processes are essential for the survival and functioning of organisms.
Action Environment
The action of porphine can be influenced by various environmental factors. For instance, the efficiency of porphine in photosynthesis (as part of chlorophyll) can be affected by the intensity and quality of light . Additionally, certain environmental toxins can affect the biosynthesis of porphyrins, leading to disorders known as porphyrias .
生化学分析
Biochemical Properties
Porphine interacts with various biomolecules, playing a significant role in biochemical reactions. It serves as an enzymatic cofactor, participating in the generation, storage, and use of oxygen, which is pivotal to life . Its photochemical properties are central to the biochemical functioning of plants .
Cellular Effects
Porphine and its derivatives have profound effects on various types of cells and cellular processes. For instance, porphyrins, when complexed to metals, can engage in a multitude of contemporary applications ranging from solar energy generation to serving as catalysts for important chemical reactions
Molecular Mechanism
The molecular mechanism of porphine involves its planar, aromatic structure, which consists of four pyrrole-like rings interconnected by methine bridges . This structure allows porphine to absorb strongly in the visible region of the electromagnetic spectrum, making it deeply colored
Temporal Effects in Laboratory Settings
One study found that porphine showed excellent coupling with a graphene electrode, suggesting potential stability and robustness in certain conditions .
Metabolic Pathways
Porphine is involved in several metabolic pathways due to its role as an enzymatic cofactor
Transport and Distribution
One study found that porphine showed excellent coupling with a graphene electrode, suggesting potential for transport and distribution in certain conditions .
準備方法
Synthetic Routes and Reaction Conditions: Porphine can be synthesized through several methods. One common preparation method involves heating urea, phthalic anhydride, and a copper salt (e.g., copper chloride, copper carbonate, or copper sulfate) in the presence of a molybdenum catalyst such as ammonium molybdate or molybdenum trioxide . This method can be modified using microwave-assisted synthesis or ionic liquids to improve yields and reduce reaction times .
Industrial Production Methods: Industrial production of porphine and its derivatives often involves large-scale adaptations of the aforementioned synthetic routes. The use of microwave-assisted synthesis and ionic liquids has been explored to enhance efficiency and sustainability in industrial settings .
化学反応の分析
Types of Reactions: Porphine undergoes various chemical reactions, including oxidation, reduction, and substitution reactions.
Common Reagents and Conditions:
Oxidation: Porphine can be oxidized using reagents such as hydrogen peroxide or potassium permanganate under acidic conditions.
Reduction: Reduction of porphine can be achieved using reducing agents like sodium borohydride or lithium aluminum hydride.
Substitution: Substitution reactions often involve halogenation or nitration using reagents like chlorine or nitric acid.
Major Products: The major products formed from these reactions include hydroxyporphine derivatives from oxidation, reduced porphine species from reduction, and halogenated or nitrated porphine derivatives from substitution reactions .
類似化合物との比較
Protoporphyrin IX: A naturally occurring porphyrin that serves as a precursor to heme.
Octaethylporphyrin: A synthetic analogue of protoporphyrin IX, known for its high symmetry.
Tetraphenylporphyrin: Another synthetic analogue with phenyl groups at the methine centers.
Uniqueness: Porphine is unique due to its simple structure, which serves as the foundational framework for more complex porphyrins. Its ability to form stable metal complexes and participate in essential biological processes highlights its significance in both natural and synthetic chemistry .
生物活性
Porphine is a cyclic compound that serves as a precursor to various porphyrins, which are essential in biological systems due to their role in oxygen transport, photosynthesis, and electron transfer. This article explores the biological activities of porphine, particularly its antimicrobial, antiviral, and anticancer properties, supported by case studies and research findings.
1. Antimicrobial Activity
Porphine and its derivatives exhibit significant antimicrobial properties. Research indicates that porphyrin compounds can catalyze peroxidase and oxidase reactions, generating reactive oxygen species (ROS) that damage bacterial membranes. This light-dependent photodynamic activity has been demonstrated against both Gram-positive and Gram-negative bacteria. Notably, non-iron metalloporphyrins show light-independent antimicrobial effects by enhancing bacterial sensitivity to ROS or directly producing ROS .
Table 1: Antimicrobial Activity of Porphine Derivatives
Compound Type | Mechanism of Action | Target Organisms |
---|---|---|
Natural Porphyrins | Photodynamic action | Various bacteria |
Non-Iron Metalloporphyrins | ROS generation | Gram-positive and Gram-negative |
Synthetic Porphyrins | Antibiotic conjugates | Pathogenic microorganisms |
2. Antiviral Activity
Porphine has also been investigated for its antiviral properties, particularly against enveloped viruses like HIV and SARS-CoV-2. Studies show that porphyrin compounds can inhibit various stages of the HIV life cycle by interacting with viral proteins, such as inhibiting reverse transcriptase and HIV protease . The binding interactions between porphine and viral components can disrupt critical functions necessary for viral replication.
Case Study: Inhibition of SARS-CoV-2
A recent study analyzed the interaction of porphine with the nucleocapsid protein of SARS-CoV-2. The results indicated that porphine could bind effectively to the dimerization region of the nucleocapsid protein, potentially inhibiting viral propagation . The binding energy was measured at -8.6 kcal/mol, suggesting a strong interaction.
Table 2: Antiviral Efficacy of Porphine
Virus | Mechanism of Action | Effective Concentration (μg/mL) | Observed Effect |
---|---|---|---|
HIV | RT inhibition | 6-10 | 75% CPE reduction |
SARS-CoV-2 | Nucleocapsid protein binding | 4-10 | Inhibition of viral replication |
3. Anticancer Properties
Porphine has shown promise in cancer treatment, particularly in targeting liver cancer. A case-control study involving patients with acute intermittent porphyria (AIP) revealed that elevated levels of porphyrin precursors were associated with an increased risk of primary liver cancer . This suggests a complex relationship where porphyrin metabolism may influence cancer risk.
Research Findings: Urinary Porphyrin Levels in AIP Patients
The study analyzed urinary excretion levels of porphyrin precursors (5-aminolevulinic acid and porphobilinogen) in AIP patients who developed liver cancer compared to controls. The findings indicated significantly higher levels in those who developed cancer, highlighting the potential role of porphyrins in oncogenesis.
Table 3: Urinary Porphyrin Levels in AIP Patients
Group | Median PBG (mmol/mol creatinine) | Odds Ratio (95% CI) |
---|---|---|
Patients with PLC | 7.9 (IQR 4.4-21.9) | 1.07 (1.02-1.12) |
Controls without PLC | 3.8 (IQR 1.2-9.8) | - |
特性
IUPAC Name |
21,22-dihydroporphyrin | |
---|---|---|
Source | PubChem | |
URL | https://pubchem.ncbi.nlm.nih.gov | |
Description | Data deposited in or computed by PubChem | |
InChI |
InChI=1S/C20H14N4/c1-2-14-10-16-5-6-18(23-16)12-20-8-7-19(24-20)11-17-4-3-15(22-17)9-13(1)21-14/h1-12,21-22H | |
Source | PubChem | |
URL | https://pubchem.ncbi.nlm.nih.gov | |
Description | Data deposited in or computed by PubChem | |
InChI Key |
JZRYQZJSTWVBBD-UHFFFAOYSA-N | |
Source | PubChem | |
URL | https://pubchem.ncbi.nlm.nih.gov | |
Description | Data deposited in or computed by PubChem | |
Canonical SMILES |
C1=CC2=CC3=CC=C(N3)C=C4C=CC(=N4)C=C5C=CC(=N5)C=C1N2 | |
Source | PubChem | |
URL | https://pubchem.ncbi.nlm.nih.gov | |
Description | Data deposited in or computed by PubChem | |
Molecular Formula |
C20H14N4 | |
Source | PubChem | |
URL | https://pubchem.ncbi.nlm.nih.gov | |
Description | Data deposited in or computed by PubChem | |
DSSTOX Substance ID |
DTXSID6059235 | |
Record name | 21H,23H-Porphine | |
Source | EPA DSSTox | |
URL | https://comptox.epa.gov/dashboard/DTXSID6059235 | |
Description | DSSTox provides a high quality public chemistry resource for supporting improved predictive toxicology. | |
Molecular Weight |
310.4 g/mol | |
Source | PubChem | |
URL | https://pubchem.ncbi.nlm.nih.gov | |
Description | Data deposited in or computed by PubChem | |
CAS No. |
101-60-0 | |
Record name | Porphine | |
Source | ChemIDplus | |
URL | https://pubchem.ncbi.nlm.nih.gov/substance/?source=chemidplus&sourceid=0000101600 | |
Description | ChemIDplus is a free, web search system that provides access to the structure and nomenclature authority files used for the identification of chemical substances cited in National Library of Medicine (NLM) databases, including the TOXNET system. | |
Record name | 21H,23H-Porphine | |
Source | EPA Chemicals under the TSCA | |
URL | https://www.epa.gov/chemicals-under-tsca | |
Description | EPA Chemicals under the Toxic Substances Control Act (TSCA) collection contains information on chemicals and their regulations under TSCA, including non-confidential content from the TSCA Chemical Substance Inventory and Chemical Data Reporting. | |
Record name | 21H,23H-Porphine | |
Source | EPA DSSTox | |
URL | https://comptox.epa.gov/dashboard/DTXSID6059235 | |
Description | DSSTox provides a high quality public chemistry resource for supporting improved predictive toxicology. | |
Record name | 21H,23H-porphine | |
Source | European Chemicals Agency (ECHA) | |
URL | https://echa.europa.eu/substance-information/-/substanceinfo/100.002.690 | |
Description | The European Chemicals Agency (ECHA) is an agency of the European Union which is the driving force among regulatory authorities in implementing the EU's groundbreaking chemicals legislation for the benefit of human health and the environment as well as for innovation and competitiveness. | |
Explanation | Use of the information, documents and data from the ECHA website is subject to the terms and conditions of this Legal Notice, and subject to other binding limitations provided for under applicable law, the information, documents and data made available on the ECHA website may be reproduced, distributed and/or used, totally or in part, for non-commercial purposes provided that ECHA is acknowledged as the source: "Source: European Chemicals Agency, http://echa.europa.eu/". Such acknowledgement must be included in each copy of the material. ECHA permits and encourages organisations and individuals to create links to the ECHA website under the following cumulative conditions: Links can only be made to webpages that provide a link to the Legal Notice page. | |
Record name | PORPHINE | |
Source | FDA Global Substance Registration System (GSRS) | |
URL | https://gsrs.ncats.nih.gov/ginas/app/beta/substances/604BGD9J5B | |
Description | The FDA Global Substance Registration System (GSRS) enables the efficient and accurate exchange of information on what substances are in regulated products. Instead of relying on names, which vary across regulatory domains, countries, and regions, the GSRS knowledge base makes it possible for substances to be defined by standardized, scientific descriptions. | |
Explanation | Unless otherwise noted, the contents of the FDA website (www.fda.gov), both text and graphics, are not copyrighted. They are in the public domain and may be republished, reprinted and otherwise used freely by anyone without the need to obtain permission from FDA. Credit to the U.S. Food and Drug Administration as the source is appreciated but not required. | |
Retrosynthesis Analysis
AI-Powered Synthesis Planning: Our tool employs the Template_relevance Pistachio, Template_relevance Bkms_metabolic, Template_relevance Pistachio_ringbreaker, Template_relevance Reaxys, Template_relevance Reaxys_biocatalysis model, leveraging a vast database of chemical reactions to predict feasible synthetic routes.
One-Step Synthesis Focus: Specifically designed for one-step synthesis, it provides concise and direct routes for your target compounds, streamlining the synthesis process.
Accurate Predictions: Utilizing the extensive PISTACHIO, BKMS_METABOLIC, PISTACHIO_RINGBREAKER, REAXYS, REAXYS_BIOCATALYSIS database, our tool offers high-accuracy predictions, reflecting the latest in chemical research and data.
Strategy Settings
Precursor scoring | Relevance Heuristic |
---|---|
Min. plausibility | 0.01 |
Model | Template_relevance |
Template Set | Pistachio/Bkms_metabolic/Pistachio_ringbreaker/Reaxys/Reaxys_biocatalysis |
Top-N result to add to graph | 6 |
Feasible Synthetic Routes
試験管内研究製品の免責事項と情報
BenchChemで提示されるすべての記事および製品情報は、情報提供を目的としています。BenchChemで購入可能な製品は、生体外研究のために特別に設計されています。生体外研究は、ラテン語の "in glass" に由来し、生物体の外で行われる実験を指します。これらの製品は医薬品または薬として分類されておらず、FDAから任何の医療状態、病気、または疾患の予防、治療、または治癒のために承認されていません。これらの製品を人間または動物に体内に導入する形態は、法律により厳格に禁止されています。これらのガイドラインに従うことは、研究と実験において法的および倫理的な基準の遵守を確実にするために重要です。