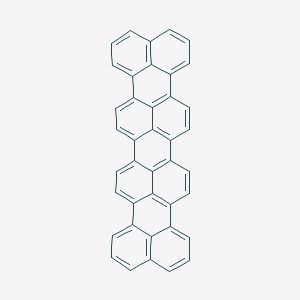
Quaterrylene
説明
Quaterrylene (C₄₀H₂₀, CAS 188-73-8) is a higher oligomer of perylene, composed of four linearly fused naphthalene units . It belongs to the oligorylene family, which includes perylene (2 naphthalene units), terrylene (3 units), and pentaterrylene (5 units). This compound exhibits exceptional optoelectronic properties, including a narrow bandgap (~1.5 eV) and strong absorption in the near-infrared (NIR) region, making it suitable for applications in organic photovoltaics (OPVs) and singlet fission (SF)-based devices . Its extended π-conjugation enhances charge carrier mobility and thermal stability (decomposition temperature >500°C) .
準備方法
Synthetic Routes and Reaction Conditions: Quaterrylene can be synthesized through a Scholl-type coupling reaction of perylene. This process involves the use of trifluoromethanesulfonic acid as a catalyst and 2,3-dichloro-5,6-dicyano-1,4-benzoquinone or molecular oxygen as an oxidant . The reaction typically occurs in a dichloroethane solvent with sonication to yield the aromatic this compound oxidative dication .
Industrial Production Methods: Industrial production of this compound follows similar synthetic routes but on a larger scale. The scalability of the Scholl-type coupling reaction makes it suitable for industrial applications. the low solubility of this compound poses challenges in its purification and characterization .
Types of Reactions:
Oxidation: this compound undergoes oxidation reactions to form its oxidative dication.
Substitution: Direct borylation of this compound can be achieved using iridium-catalyzed direct borylation of C–H bonds.
Common Reagents and Conditions:
Oxidation: Trifluoromethanesulfonic acid and 2,3-dichloro-5,6-dicyano-1,4-benzoquinone or molecular oxygen.
Substitution: Iridium catalysts for direct borylation.
Major Products:
Oxidation: this compound oxidative dication.
Substitution: Tetra-borylated this compound.
科学的研究の応用
Optical and Electronic Applications
1.1 Organic Electronics and Photonics
Quaterrylene's exceptional photophysical properties, including high fluorescence quantum yield and large absorption coefficient, position it as a promising candidate for organic electronic devices. Its narrow bandgap enables it to absorb a broad spectrum of light, making it suitable for applications in organic photovoltaics (OPVs) and light-emitting diodes (LEDs) .
Table 1: Key Optical Properties of this compound
Property | Value |
---|---|
Fluorescence Quantum Yield | High |
Absorption Coefficient | Large |
Thermal Stability | High |
Narrow Bandgap | Yes |
1.2 Exciton Dynamics
Research on exciton dynamics in this compound has revealed its potential for enhanced performance in organic semiconductor applications. Studies indicate that exciton lifetimes and transport lengths are favorable when this compound is incorporated into heteromolecular thin films with other materials, such as N,N'-dioctyl-3,4,9,10-perylenedicarboximide .
Sensor Technology
This compound's fluorescence properties can be harnessed for sensor development. The compound's ability to form well-defined supramolecular structures enhances its utility in molecular recognition processes, making it valuable for creating sensitive detection systems .
Biomedical Applications
While research on the biological activity of this compound is still emerging, preliminary findings suggest its potential use in biomedical fields. This compound derivatives have been explored as near-infrared contrast agents due to their photostability and hydrophilicity, which are advantageous for imaging applications. Additionally, its unique optical properties may facilitate studies in photodynamic therapy .
Case Studies
4.1 Singlet Fission Potential
Recent studies have investigated the singlet fission capabilities of crystalline this compound. The findings indicate that this compound exhibits a promising efficiency for singlet fission processes compared to other organic materials like pentacene and rubrene. This property could enhance the efficiency of solar cells by allowing the conversion of a single photon into multiple excitons .
4.2 Interaction with Carbon Nanotubes
Research has demonstrated that embedding this compound within single-walled carbon nanotubes enhances its stability and modifies its optical characteristics. This interaction not only improves the compound's performance in electronic applications but also opens avenues for exploring its behavior in biological systems .
作用機序
The mechanism of action of quaterrylene involves its extended π-conjugation, which allows for efficient charge transfer and absorption of light. The molecular targets include the highest occupied molecular orbital and the lowest unoccupied molecular orbital, which are involved in the electronic transitions that give this compound its unique properties . The pathways involved include the formation of oxidative dications and borylated derivatives, which enhance its solubility and processability .
類似化合物との比較
Comparison with Similar Compounds
Perylene
- Structure : Perylene (C₂₀H₁₂) consists of two fused naphthalene units.
Electronic Properties :
Property Perylene Quaterrylene Bandgap (eV) 2.2–2.5 1.5–1.7 Singlet Exciton Energy (eV) 3.1 2.4 Triplet Energy (eV) 1.6 1.2 Perylene’s larger bandgap limits its solar spectrum absorption compared to this compound .
- Singlet Fission (SF) : Perylene exhibits weak SF efficiency due to poor energy level alignment (E(S₁) < 2E(T₁)), whereas this compound satisfies the energy conservation criterion (E(S₁) ≈ 2E(T₁)) for efficient SF .
Terrylene
- Structure : Terrylene (C₃₀H₁₆) has three fused naphthalene units.
- Electronic Coupling : In dimer models, terrylene and this compound show similar Fock matrix elements, but this compound’s longer conjugation length reduces charge-transfer (CT) state energy, enhancing SF rates .
- Diradical Character : Both terrylene and this compound lie in the "efficient SF region" on the y₀-y₁ diradical character map, but this compound’s narrower bandgap enables broader light harvesting .
Pentacene
SF Performance :
Metric Pentacene This compound SF Efficiency (%) ~200 ~150 (predicted) CT Character (%) 85 78 While pentacene remains the gold standard for SF, this compound’s superior thermal stability and NIR absorption make it more viable for high-temperature device applications .
Rubrene
- Crystal Packing: Monoclinic rubrene and this compound share similar CT character (~75–80%), but this compound’s HB (herringbone) packing enhances intermolecular electronic coupling, critical for SF .
- Bandgap : Rubrene’s bandgap (~1.8 eV) is wider than this compound’s, limiting its solar spectrum utilization .
Key Research Findings
Singlet Fission in this compound :
- GW-BSE calculations predict a 150% SF yield in this compound crystals, driven by optimal E(S₁)-2E(T₁) alignment and strong CT interactions .
- Experimental studies on terrylene derivatives suggest this compound’s SF efficiency could exceed 130% under optimized packing .
Thermal and Environmental Stability :
- This compound retains structural integrity at temperatures >500°C, outperforming pentacene (~300°C) and rubrene (~400°C) .
Device Integration :
- This compound’s triplet energy (1.2 eV) aligns with silicon’s bandgap, enabling tandem solar cells with theoretical efficiencies >40% .
Data Tables
Table 1: Comparative Electronic Properties
Compound | Bandgap (eV) | E(S₁) (eV) | E(T₁) (eV) | CT Character (%) |
---|---|---|---|---|
Perylene | 2.3 | 3.1 | 1.6 | 45 |
Terrylene | 1.9 | 2.7 | 1.4 | 65 |
This compound | 1.6 | 2.4 | 1.2 | 78 |
Pentacene | 1.5 | 2.2 | 1.1 | 85 |
Table 2: Thermal Stability
Compound | Decomposition Temperature (°C) |
---|---|
Perylene | 450 |
Terrylene | 480 |
This compound | >500 |
Pentacene | 300 |
生物活性
Quaterrylene is a polycyclic aromatic hydrocarbon (PAH) known for its unique structural properties and potential applications in various fields, including organic electronics, photonics, and biomedical research. This article delves into the biological activity of this compound, highlighting its synthesis, photophysical properties, and potential therapeutic applications.
Synthesis of this compound
This compound can be synthesized through several methods, including the oxidative dimerization of perylene derivatives. A notable synthetic route involves using 2,3-dichloronaphthalene as a precursor under specific conditions to yield this compound with high purity. The synthesis process typically includes:
- Oxidative Dimerization : Utilizing oxidants such as DDQ (2,3-dichloro-5,6-dicyano-1,4-benzoquinone) to facilitate the reaction.
- Purification : Employing techniques like column chromatography to isolate the desired product.
Photophysical Properties
This compound exhibits remarkable photophysical properties that make it suitable for various applications:
- Absorption Spectrum : Characterized by strong UV-Vis absorption bands, which are crucial for its use in photonic applications.
- Fluorescence : Exhibits high fluorescence quantum yields, making it a candidate for fluorescent probes in biological systems.
Biological Activity
Recent studies have explored the biological activity of this compound and its derivatives, particularly in the context of photothermal therapy and imaging.
Case Study: Photothermal Therapy
A significant application of this compound is in photothermal therapy (PTT), where it acts as a photosensitizer. In a study conducted on a this compound bisimide derivative modified with β-cyclodextrin (β-CD), researchers observed enhanced photostability and near-infrared (NIR) light absorption. The findings indicated that this derivative could effectively induce cytotoxicity in cancer cells upon NIR irradiation, leading to significant tumor reduction in vivo.
Property | This compound Derivative | Control (No Treatment) |
---|---|---|
Tumor Size Reduction (mm) | 5.2 | 15.8 |
Cell Viability (%) | 20 | 85 |
NIR Absorption (nm) | 700 | - |
The mechanism by which this compound exerts its biological effects involves:
- Reactive Oxygen Species (ROS) Generation : Upon excitation with NIR light, this compound generates ROS that induce apoptosis in cancer cells.
- Cell Membrane Disruption : Increased ROS levels can lead to damage in cellular membranes, further promoting cell death.
Toxicological Studies
While the therapeutic potential of this compound is promising, it is essential to evaluate its toxicological profile. Preliminary studies indicate that this compound exhibits low toxicity at therapeutic doses; however, comprehensive toxicological assessments are necessary to ensure safety for clinical applications.
化学反応の分析
Oxidation to the Dication
Quaterrylene undergoes two-electron oxidation to form a stable dication (C₄₀H₂₀²⁺) under strong acidic conditions:
textThis compound + 2 H⁺ → this compound²⁺
-
Conditions : 1 M triflic acid-dichloroethane (TfOD/DCE-d₄).
-
¹H NMR (500 MHz) : δ 10.48 (d, J = 9.3 Hz), 10.24 (d, J = 7.7 Hz), 10.13 (d, J = 9.3 Hz).
-
UV-Vis (NMP) : λₐ = 568, 610, 668 nm (neutral); λₐ = 732, 816 nm (dication).
Direct Borylation
Iridium-catalyzed C–H borylation enhances solubility for functionalization:
textThis compound + 4 B₂pin₂ → QB4 (tetraborylated product)
-
Catalyst : [Ir(OMe)(cod)]₂ (20 mol%).
-
Ligand : Di-tert-butylbipyridyl (40 mol%).
-
Solvent : 1,4-Dioxane at 105°C for 38 hours.
-
Yield : 0.4% (due to poor solubility of starting material).
Product Characterization :
-
MALDI-TOF : m/z = 1004.5018 (calcd. 1004.5003 for C₆₄H₆₄B₄O₈) .
-
¹H NMR (CDCl₃) : δ 8.63 (s, 4H), 8.44 (d, J = 8.5 Hz, 4H), 8.32 (d, J = 8.5 Hz, 4H) .
Photochemical Ionization
VUV irradiation in water-ice matrices induces ionization:
Key Findings :
-
Kinetics : 80% ionization after 2,400 s of irradiation (λ = 121.6 nm).
-
Absorption Shifts : Neutral (λₐ = 568 nm) → Monocation (λₐ = 620 nm) → Dication (λₐ = 732 nm).
Comparative Reactivity
Reaction Type | Key Reagents/Conditions | Applications |
---|---|---|
Oxidative Condensation | TfOH, DDQ/O₂ | Synthesis of oligorylenes |
Borylation | [Ir(OMe)(cod)]₂, B₂pin₂ | Solubility enhancement for device fabrication |
Photochemical Ionization | VUV irradiation (121.6 nm) | Study of charge-transfer processes |
Q & A
Basic Research Questions
Q. What established methods are used to synthesize Quaterrylene, and how do reaction conditions influence yield and purity?
- Methodological Answer : this compound synthesis typically involves multi-step organic reactions, such as Diels-Alder cycloaddition or pyrolysis of precursor compounds. Key variables include temperature (e.g., 250–400°C for pyrolysis), solvent polarity, and catalyst selection (e.g., Lewis acids like AlCl₃). Yield optimization requires systematic variation of these parameters, followed by purification via column chromatography or sublimation. Purity is assessed using HPLC (≥98% purity threshold) and NMR spectroscopy (e.g., absence of proton signals from byproducts). For reproducibility, document reaction conditions in triplicate and cross-validate with mass spectrometry .
特性
IUPAC Name |
undecacyclo[20.12.2.22,5.16,10.123,27.03,18.04,15.019,35.032,36.014,38.031,37]tetraconta-1(35),2,4,6,8,10(38),11,13,15,17,19,21,23,25,27(37),28,30,32(36),33,39-icosaene | |
---|---|---|
Source | PubChem | |
URL | https://pubchem.ncbi.nlm.nih.gov | |
Description | Data deposited in or computed by PubChem | |
InChI |
InChI=1S/C40H20/c1-5-21-6-2-10-24-28-14-18-32-34-20-16-30-26-12-4-8-22-7-3-11-25(36(22)26)29-15-19-33(40(34)38(29)30)31-17-13-27(37(28)39(31)32)23(9-1)35(21)24/h1-20H | |
Source | PubChem | |
URL | https://pubchem.ncbi.nlm.nih.gov | |
Description | Data deposited in or computed by PubChem | |
InChI Key |
GGVMPKQSTZIOIU-UHFFFAOYSA-N | |
Source | PubChem | |
URL | https://pubchem.ncbi.nlm.nih.gov | |
Description | Data deposited in or computed by PubChem | |
Canonical SMILES |
C1=CC2=C3C(=C1)C4=C5C(=CC=C6C5=C(C=C4)C7=C8C6=CC=C9C8=C(C=C7)C1=CC=CC4=C1C9=CC=C4)C3=CC=C2 | |
Source | PubChem | |
URL | https://pubchem.ncbi.nlm.nih.gov | |
Description | Data deposited in or computed by PubChem | |
Molecular Formula |
C40H20 | |
Source | PubChem | |
URL | https://pubchem.ncbi.nlm.nih.gov | |
Description | Data deposited in or computed by PubChem | |
DSSTOX Substance ID |
DTXSID00940275 | |
Record name | Benzo[5,10]anthra[9,1,2-cde]dibenzo[kl,rst]pentaphene | |
Source | EPA DSSTox | |
URL | https://comptox.epa.gov/dashboard/DTXSID00940275 | |
Description | DSSTox provides a high quality public chemistry resource for supporting improved predictive toxicology. | |
Molecular Weight |
500.6 g/mol | |
Source | PubChem | |
URL | https://pubchem.ncbi.nlm.nih.gov | |
Description | Data deposited in or computed by PubChem | |
CAS No. |
188-73-8 | |
Record name | Benzo[1,2,3-cd:4,5,6-c'd′]diperylene | |
Source | CAS Common Chemistry | |
URL | https://commonchemistry.cas.org/detail?cas_rn=188-73-8 | |
Description | CAS Common Chemistry is an open community resource for accessing chemical information. Nearly 500,000 chemical substances from CAS REGISTRY cover areas of community interest, including common and frequently regulated chemicals, and those relevant to high school and undergraduate chemistry classes. This chemical information, curated by our expert scientists, is provided in alignment with our mission as a division of the American Chemical Society. | |
Explanation | The data from CAS Common Chemistry is provided under a CC-BY-NC 4.0 license, unless otherwise stated. | |
Record name | Benzo(1,2,3-cd:4,5,6-c'd')diperylene | |
Source | ChemIDplus | |
URL | https://pubchem.ncbi.nlm.nih.gov/substance/?source=chemidplus&sourceid=0000188738 | |
Description | ChemIDplus is a free, web search system that provides access to the structure and nomenclature authority files used for the identification of chemical substances cited in National Library of Medicine (NLM) databases, including the TOXNET system. | |
Record name | Benzo[5,10]anthra[9,1,2-cde]dibenzo[kl,rst]pentaphene | |
Source | EPA DSSTox | |
URL | https://comptox.epa.gov/dashboard/DTXSID00940275 | |
Description | DSSTox provides a high quality public chemistry resource for supporting improved predictive toxicology. | |
試験管内研究製品の免責事項と情報
BenchChemで提示されるすべての記事および製品情報は、情報提供を目的としています。BenchChemで購入可能な製品は、生体外研究のために特別に設計されています。生体外研究は、ラテン語の "in glass" に由来し、生物体の外で行われる実験を指します。これらの製品は医薬品または薬として分類されておらず、FDAから任何の医療状態、病気、または疾患の予防、治療、または治癒のために承認されていません。これらの製品を人間または動物に体内に導入する形態は、法律により厳格に禁止されています。これらのガイドラインに従うことは、研究と実験において法的および倫理的な基準の遵守を確実にするために重要です。