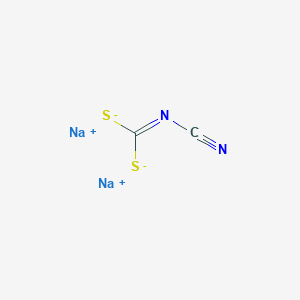
Disodium cyanodithioimidocarbonate
概要
説明
Disodium cyanodithioimidocarbonate (CAS 138-93-2) is an organosulfur compound with the molecular formula C₂N₂S₂Na₂. It belongs to the cyanodithioimidocarbonate family, characterized by a dithiocarbamate-like backbone with a cyano group and two sulfur atoms bound to a central carbon-nitrogen core. The compound is primarily used as a biocide in industrial water treatment systems, particularly in cooling towers, where it inhibits microbial growth at alkaline pH (>7.5) . Its reactivity stems from the [C₂N₂S₂]²⁻ ligand, which can coordinate with transition metals to form complexes with unique conducting properties .
準備方法
Disodium cyanodithioimidocarbonate can be synthesized through the reaction of cyanamide with carbon disulfide in the presence of an alkali metal hydroxide in aqueous ethanol. The intermediate dialkali metal salt of N-cyanodithioiminocarbonic acid is then alkylated with methyl iodide or dimethyl sulfate . Industrial production methods involve formulating the compound as a soluble concentrate or liquid, which is then applied using measuring containers, metering pumps, or drip-feed devices .
化学反応の分析
Hydrolytic Decomposition in Aqueous Media
DCDIC undergoes hydrolysis in water, with decomposition accelerated under acidic conditions. The primary products include carbon disulfide (CS₂) and methylamine (CH₃NH₂), as observed in studies of dithiocarbamate derivatives .
Reaction Conditions | Products | Mechanism |
---|---|---|
Neutral pH, 25°C | Slow decomposition to CS₂ + amines | Base-catalyzed hydrolysis |
Acidic conditions (pH < 4) | Rapid release of CS₂ + NH₃/CH₃NH₂ | Acid-promoted breakdown of thiocarbamate bonds |
This reactivity necessitates careful pH control during industrial applications to prevent premature degradation .
Reactions with Acids and Oxidizers
DCDIC reacts violently with strong acids (e.g., H₂SO₄) and oxidizing agents (e.g., peroxides), generating toxic gases such as hydrogen sulfide (H₂S) and methyl isothiocyanate (CH₃NCS) .
Key Observations:
Nucleophilic Substitution Reactions
The methylthio (-SMe) and cyano (-CN) groups in DCDIC are susceptible to nucleophilic attack, enabling heterocycle synthesis.
3.1. Reaction with Amines
Primary amines (e.g., methylamine) displace methylthio groups, forming substituted isothioureas. For example:
These intermediates cyclize to triazoles or pyrimidines under basic conditions .
3.2. Cyclocondensation with Hydrazines
Hydrazine (N₂H₄) reacts with DCDIC to yield 3,5-diaminotriazoles (e.g., 67 , 68 ) or oxadiazoles (e.g., 69 ), depending on the nucleophile :
Nucleophile | Product | Yield |
---|---|---|
Hydrazine | 3,5-Diaminotriazole | 63–89% |
Hydroxylamine | 3,5-Diaminooxadiazole | 75% |
Organometallic Interactions
DCDIC reacts with organotin chlorides (e.g., R₃SnCl) to form tin-dithiocarbamate complexes. A 1969 study demonstrated that the cyanodithioimidocarbonate anion displaces chloride in organotin substrates, producing stable adducts :
These complexes exhibit applications in catalysis and material science .
Oxygenation Reactions
In the presence of cupric chloride (CuCl₂) and peroxydicarbonates, DCDIC participates in the oxygenation of aromatic hydrocarbons (e.g., p-xylene). The reaction generates hydroxylated derivatives via radical intermediates, with oxygen-18 isotopic tracing confirming the peroxide-mediated pathway .
科学的研究の応用
Chemical Properties and Mechanism of Action
DCDIC is characterized by its ability to act as an industrial biocide and slimicide , primarily used to control microbial growth in water treatment systems. It is soluble in water and decomposes in aqueous solutions to produce carbon disulfide and methylamine, with the decomposition accelerated by acidic conditions . Its chemical structure allows it to react with various compounds, generating flammable gases when combined with aldehydes, nitrides, or hydrides .
Industrial Applications
- Water Treatment : DCDIC is widely used in industrial water treatment systems to manage slime-forming bacteria, algae, and fungi. Its effectiveness in controlling microbial growth makes it essential in sectors such as:
- Agricultural Uses : DCDIC has been registered for use as a pesticide under the Federal Insecticide, Fungicide, and Rodenticide Act (FIFRA). Its applications include:
Scientific Research Applications
DCDIC serves as a precursor in synthesizing various heterocyclic compounds, which are crucial for advancing chemical research. Notable applications include:
- Synthesis of Cyanoguanidines : DCDIC is utilized in creating cyanoguanidines, which have potential applications in pharmaceuticals.
- Protein Tyrosine Kinase Inhibitors : Derivatives of DCDIC have shown promise as inhibitors for protein tyrosine kinases, which are significant targets in cancer therapy.
- GABA-B Modulators : Compounds derived from DCDIC exhibit activity as GABA-B receptor modulators, indicating potential neurological applications .
Case Study 1: Efficacy in Water Treatment
A study conducted on the effectiveness of DCDIC in cooling water systems demonstrated a significant reduction in microbial load when treated with DCDIC compared to untreated systems. The results indicated that DCDIC maintained a residual effect that inhibited regrowth of bacteria over extended periods.
Parameter | Untreated System | DCDIC Treated System |
---|---|---|
Initial Bacterial Count (CFU/mL) | 1.5 x 10^6 | 2.0 x 10^3 |
Bacterial Count after 7 Days (CFU/mL) | 2.0 x 10^7 | 1.0 x 10^2 |
Case Study 2: Agricultural Application
In a field trial assessing the effectiveness of DCDIC as a nematicide, crops treated with DCDIC exhibited a 30% increase in yield compared to untreated controls due to reduced nematode populations.
Crop Type | Yield (kg/ha) Untreated | Yield (kg/ha) DCDIC Treated |
---|---|---|
Tomato | 20 | 26 |
Potato | 25 | 32 |
Safety and Environmental Considerations
While DCDIC is effective as a biocide and pesticide, it poses certain health hazards including skin and eye irritation. It is classified under acute toxic substances; hence, appropriate safety measures must be implemented during handling and application . Regulatory assessments by agencies such as the Environmental Protection Agency (EPA) have evaluated its environmental impact and established guidelines for safe usage .
作用機序
The mechanism of action of disodium cyanodithioimidocarbonate involves its decomposition in aqueous solutions to form carbon disulfide and methylamine or other amines. This decomposition process is accelerated by acids . The compound’s biocidal activity is attributed to its ability to disrupt microbial cell membranes and inhibit essential enzymatic processes .
類似化合物との比較
Structural Analogs
Dimethyl N-Cyanodithioiminocarbonate
- CAS: Not explicitly listed in evidence.
- Molecular Formula : C₄H₆N₂S₂ (inferred from methyl substitution).
- Applications: Primarily used in organic synthesis to construct fused heterocycles with biological activity (e.g., antimicrobial, anticancer agents) .
- Key Differences : The methyl groups render it more lipophilic, favoring organic-phase reactions. Its reactivity in heterocycle synthesis highlights its role as a precursor in medicinal chemistry, contrasting with the disodium salt’s industrial biocidal use .
Dipotassium Cyanodithioimidocarbonate
- CAS: Not explicitly provided, but referenced in coordination chemistry studies .
- Molecular Formula : C₂N₂S₂K₂ (inferred from potassium substitution).
- Applications: Limited evidence, but likely shares biocidal properties with the disodium analog.
- Key Differences : The choice of counterion (Na⁺ vs. K⁺) may influence solubility and coordination behavior in metal complexes .
Functional Analogs
Potassium N-Methyldithiocarbamate
- CAS: Not explicitly listed.
- Molecular Formula : C₃H₆NS₂K (inferred).
- Applications: Often combined with disodium cyanodithioimidocarbonate in biocidal formulations (e.g., Wemicde CW 104 and Bulab 6003) to enhance efficacy against microbial populations in cooling towers .
- Key Differences: Structurally distinct as a dithiocarbamate (lacking the cyano group), it operates via a different mechanism, likely disrupting microbial enzyme systems. Both compounds, however, share acute toxicity profiles (skin/eye irritation, aquatic toxicity) .
Diuron (CAS 330-54-1)
- Molecular Formula : C₉H₁₀Cl₂N₂O.
- Applications: A urea-based herbicide listed alongside this compound in EPA regulatory documents . Unlike the latter, it inhibits photosynthesis in plants and lacks biocidal activity in water systems.
- Key Differences: Highlights the diversity in functional groups (urea vs. cyanodithioimidocarbonate) driving distinct environmental and biological interactions.
Data Tables
Table 1: Structural and Functional Comparison
Table 2: Toxicity and Regulatory Status
生物活性
Disodium cyanodithioimidocarbonate (DCDIC), a chemical compound primarily used as an industrial biocide and slimicide, has been studied for its biological activity, particularly in controlling microbial growth in various settings, including food processing and industrial water systems. This article provides an overview of its biological activity, including its mechanisms of action, toxicity profiles, and relevant case studies.
Chemical Overview
DCDIC is classified as a microbicide/microbistat, effective against slime-forming bacteria, algae, and fungi. Its chemical structure allows it to disrupt cellular processes in microorganisms, making it valuable in industrial applications where microbial contamination can pose significant operational challenges.
The primary mechanism of action for DCDIC involves interference with microbial cellular processes. It is believed to inhibit the synthesis of essential biomolecules or disrupt cellular membranes, leading to cell death. This biocidal activity is particularly effective in aqueous environments where biofilms can form.
Acute Toxicity
Research indicates that DCDIC exhibits moderate acute toxicity. The Environmental Protection Agency (EPA) has classified it based on various studies that assess its impact on human health and the environment. For instance:
- Oral Toxicity : Studies have shown that the compound has a median lethal dose (LD50) indicating moderate toxicity when ingested.
- Dermal Exposure : Skin contact may lead to irritation; however, significant systemic effects have not been widely reported.
Chronic Toxicity
Chronic exposure studies have raised concerns about potential reproductive and developmental effects. For example:
- Developmental Toxicity : Certain studies indicated an increased incidence of developmental abnormalities in animal models exposed to high doses of DCDIC during gestation .
- Mutagenicity : The compound has not been conclusively shown to be mutagenic; however, ongoing assessments are necessary to fully understand its long-term effects .
Industrial Applications
- Food Processing : DCDIC is utilized in food processing water systems to prevent microbial growth. Its effectiveness at low concentrations makes it a preferred choice for maintaining hygiene without adversely affecting food quality .
- Water Treatment : In industrial water treatment facilities, DCDIC has demonstrated significant efficacy in controlling biofilms and reducing the need for more hazardous chemicals .
Environmental Impact
A study conducted by the EPA assessed the environmental risks associated with DCDIC usage in various applications. The findings suggested that while the compound effectively controls microbial populations, careful management is necessary to prevent adverse ecological impacts, particularly in aquatic environments where it may affect non-target organisms .
Data Table: Summary of Biological Activity and Toxicity Profiles
Parameter | Value/Description |
---|---|
Chemical Name | This compound (DCDIC) |
Primary Use | Microbicide/microbistat |
Mechanism of Action | Disruption of cellular processes |
Acute Oral LD50 | Moderate toxicity (specific values vary by study) |
Skin Irritation Potential | Moderate |
Developmental Effects | Increased incidence of abnormalities in studies |
Environmental Risks | Potential impact on aquatic ecosystems |
Q & A
Q. Basic: What methodologies are recommended for synthesizing and characterizing DCDIC in laboratory settings?
Synthesis of DCDIC requires controlled conditions due to its reactive cyanodithioimidocarbonate group. A common approach involves reacting carbon disulfide with cyanamide under alkaline conditions, followed by sodium salt formation. Characterization should include:
- FT-IR spectroscopy to confirm the presence of C≡N and C=S bonds.
- Elemental analysis to verify stoichiometry.
- X-ray crystallography for structural elucidation (if crystalline forms are obtained).
Environmental monitoring protocols from the U.S. EPA highlight the need for inert atmospheres during synthesis to prevent decomposition .
Q. Basic: What analytical techniques are validated for quantifying DCDIC in environmental matrices?
Quantification in environmental samples (e.g., water, soil) typically employs:
- High-Performance Liquid Chromatography (HPLC) paired with UV-Vis detection (λ = 250–280 nm).
- Mass spectrometry (MS) for trace-level detection, especially in complex matrices.
The U.S. Geological Survey recommends coupling these methods with solid-phase extraction to improve sensitivity and reduce matrix interference .
Q. Advanced: How can researchers investigate pH-dependent decomposition pathways and cyanide release kinetics of DCDIC?
To study decomposition:
- Design pH-controlled batch experiments (e.g., pH 3–10) with DCDIC solutions, monitoring cyanide release via ion-selective electrodes or spectrophotometric methods (e.g., König reaction).
- Use kinetic modeling (e.g., pseudo-first-order rate constants) to correlate degradation rates with pH.
Evidence of cyanide release under acidic conditions necessitates strict containment protocols to mitigate toxicity risks .
Q. Advanced: How should discrepancies in reported toxicity values (e.g., LD50) across studies be addressed?
Discrepancies may arise from variations in test organisms, exposure routes, or analytical methods. To resolve these:
- Conduct comparative meta-analyses of existing data, stratifying by experimental conditions.
- Replicate key studies under standardized protocols (e.g., OECD guidelines) to isolate confounding variables.
- Validate findings using alternative assays (e.g., in vitro cytotoxicity tests vs. in vivo models) .
Q. Basic: What safety protocols are critical when handling DCDIC in laboratory environments?
- Use fume hoods and personal protective equipment (PPE) to prevent inhalation or dermal exposure.
- Implement cyanide detection systems in workspaces due to decomposition risks.
- Follow California Proposition 65 guidelines for maximum allowable dose levels (MADLs) to ensure compliance with regulatory thresholds .
Q. Advanced: How can researchers design experiments to evaluate DCDIC’s role in catalytic or coordination chemistry?
- Employ spectroscopic techniques (e.g., NMR, EPR) to study ligand exchange dynamics in transition metal complexes.
- Conduct kinetic studies under varying temperatures and pressures to assess catalytic efficiency.
- Cross-reference findings with computational models (e.g., DFT calculations) to validate reaction mechanisms .
Q. Basic: What environmental monitoring strategies are recommended for detecting DCDIC in aquatic ecosystems?
- Deploy passive samplers (e.g., POCIS) for continuous monitoring in water bodies.
- Combine HPLC-MS/MS with isotope dilution to enhance accuracy in quantifying low concentrations.
- Prioritize sampling near agricultural or industrial sites where DCDIC is historically used .
Q. Advanced: How can mechanistic studies on DCDIC’s interactions with biological macromolecules be structured?
- Use surface plasmon resonance (SPR) or isothermal titration calorimetry (ITC) to measure binding affinities with proteins/enzymes.
- Apply molecular docking simulations to predict interaction sites.
- Validate in silico results with in vitro assays (e.g., enzyme inhibition studies) to establish biological relevance .
特性
CAS番号 |
138-93-2 |
---|---|
分子式 |
C2N2Na2S2 |
分子量 |
162.15 g/mol |
IUPAC名 |
disodium;cyanoiminomethanedithiolate |
InChI |
InChI=1S/C2H2N2S2.2Na/c3-1-4-2(5)6;;/h(H2,4,5,6);;/q;2*+1/p-2 |
InChIキー |
AZDIXEXNLJMBJO-UHFFFAOYSA-L |
SMILES |
C(#N)N=C([S-])[S-].[Na+].[Na+] |
正規SMILES |
C(#N)N=C([S-])[S-].[Na+].[Na+] |
沸点 |
109 °C |
Color/Form |
Vivid orange/strong orange liquid |
密度 |
1.2719 kg/cu m |
Key on ui other cas no. |
138-93-2 |
物理的記述 |
Disodium cyanodithioimidocarbonate is a clear to orangish liquid with distinct odor. Irritates skin and eyes. |
ピクトグラム |
Acute Toxic; Irritant; Environmental Hazard |
賞味期限 |
Stable for 14 days under the following conditions: ambient temperature (23 °C); broad spectrum light; stainless steel, copper metals and iron ions. Slight decrease in % active ingredient observed when test substance is exposed to zinc ions, with significant decrease after exposure to elevated temperatures, aluminum metal, aluminum and copper ions. |
蒸気圧 |
9.16X10-6 mm Hg @ 25 °C |
製品の起源 |
United States |
Retrosynthesis Analysis
AI-Powered Synthesis Planning: Our tool employs the Template_relevance Pistachio, Template_relevance Bkms_metabolic, Template_relevance Pistachio_ringbreaker, Template_relevance Reaxys, Template_relevance Reaxys_biocatalysis model, leveraging a vast database of chemical reactions to predict feasible synthetic routes.
One-Step Synthesis Focus: Specifically designed for one-step synthesis, it provides concise and direct routes for your target compounds, streamlining the synthesis process.
Accurate Predictions: Utilizing the extensive PISTACHIO, BKMS_METABOLIC, PISTACHIO_RINGBREAKER, REAXYS, REAXYS_BIOCATALYSIS database, our tool offers high-accuracy predictions, reflecting the latest in chemical research and data.
Strategy Settings
Precursor scoring | Relevance Heuristic |
---|---|
Min. plausibility | 0.01 |
Model | Template_relevance |
Template Set | Pistachio/Bkms_metabolic/Pistachio_ringbreaker/Reaxys/Reaxys_biocatalysis |
Top-N result to add to graph | 6 |
Feasible Synthetic Routes
試験管内研究製品の免責事項と情報
BenchChemで提示されるすべての記事および製品情報は、情報提供を目的としています。BenchChemで購入可能な製品は、生体外研究のために特別に設計されています。生体外研究は、ラテン語の "in glass" に由来し、生物体の外で行われる実験を指します。これらの製品は医薬品または薬として分類されておらず、FDAから任何の医療状態、病気、または疾患の予防、治療、または治癒のために承認されていません。これらの製品を人間または動物に体内に導入する形態は、法律により厳格に禁止されています。これらのガイドラインに従うことは、研究と実験において法的および倫理的な基準の遵守を確実にするために重要です。