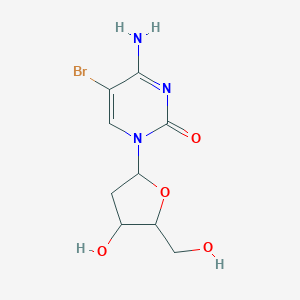
5-ブロモ-2'-デオキシシチジン
概要
説明
5-Bromo-2’-deoxycytidine is a brominated derivative of the deoxyribonucleoside 2’-deoxycytidine. It is known for its potential as a DNA photosensitizer, meaning it can induce DNA damage when exposed to light, particularly ultraviolet light . This compound has garnered interest due to its ability to form DNA cross-links and strand breaks, which can be lethal to cells .
科学的研究の応用
Molecular Biology Applications
1.1. DNA Labeling and Tracking
BrdC is widely used in molecular biology for labeling DNA, particularly in studies involving cell proliferation and differentiation. It incorporates into the DNA during the S-phase of the cell cycle, allowing researchers to trace the fate of proliferating cells. Studies have shown that BrdC can be effectively used to label neuron precursors during neurogenesis, providing insights into neuronal development and regeneration processes .
1.2. Photosensitizing Properties
Research indicates that BrdC can act as a photosensitizer when incorporated into DNA. A study demonstrated that double-stranded oligonucleotides modified with BrdC exhibited significant damage upon UVB irradiation, leading to the formation of single-strand breaks and intrastrand cross-links . This property suggests potential applications in photodynamic therapy (PDT), particularly for treating tumors in hypoxic conditions where conventional PDT may be less effective .
Cancer Research Applications
2.1. Sensitization to Radiation Therapy
BrdC has been shown to sensitize cancer cells to ionizing radiation and photoradiation. The incorporation of BrdC into DNA increases the formation of intrastrand cross-links upon exposure to radiation, which can enhance the efficacy of radiotherapy . This mechanism is particularly relevant in developing strategies for overcoming resistance in cancer treatment.
2.2. Inhibition of Tumor Growth
Studies have suggested that BrdC may inhibit tumor growth through its incorporation into DNA, leading to replication stress and cell death in rapidly dividing cancer cells . Its role as a potential therapeutic agent is being investigated in various cancer models, with promising results indicating reduced tumor burden in preclinical settings.
Table 1: Summary of Research Findings on 5-Bromo-2'-deoxycytidine
作用機序
Target of Action
The primary target of 5-Bromo-2’-deoxycytidine (BrdC) is double-stranded DNA . BrdC is incorporated into the DNA, modifying its structure .
Mode of Action
BrdC interacts with its target, the double-stranded DNA, by being incorporated into the DNA structure during the polymerase chain reaction (PCR) . This interaction results in the DNA becoming photosensitive .
Biochemical Pathways
Upon irradiation with 300 nm photons, two types of damage to the DNA are observed: single strand breaks (SSBs) and intrastrand cross-links (ICLs) . The SSBs are likely formed due to photoinduced electron transfer between the photoexcited BrdC and the ground state 2’-deoxyguanosine (dG), while the ICLs are a result of a cycloaddition reaction .
Pharmacokinetics
Its incorporation into dna during pcr suggests that it can be effectively distributed and metabolized within cells .
Result of Action
The result of BrdC’s action is the induction of potentially lethal damage to the cell . The DNA damage caused by BrdC includes SSBs and ICLs, which can lead to cell death .
Action Environment
The action of BrdC is influenced by the presence of light, specifically 300 nm photons . The DNA damage caused by BrdC is observed upon irradiation with these photons . Therefore, the efficacy and stability of BrdC’s action are likely dependent on the light conditions in its environment .
生化学分析
Biochemical Properties
5-Bromo-2’-deoxycytidine modifies double-stranded DNA and determines DNA damage when exposed to 300 nm photons . It interacts with enzymes such as polymerase during chain reaction (PCR) to label oligonucleotides .
Cellular Effects
The incorporation of 5-Bromo-2’-deoxycytidine into DNA can lead to single strand breaks (SSBs) and intrastrand cross-links (ICLs), which are potentially lethal to the cell . It has also been shown to inhibit differentiation in certain leukemia cell lines .
Molecular Mechanism
The molecular mechanism of 5-Bromo-2’-deoxycytidine involves photoinduced electron transfer between the photoexcited compound and the ground state 2’-deoxyguanosine (dG). This leads to the formation of a short-lived anion that releases the bromide anion and leaves a reactive uridine-5-yl radical in the DNA .
Temporal Effects in Laboratory Settings
In laboratory settings, the effects of 5-Bromo-2’-deoxycytidine can be observed over time. When irradiated with 300 nm photons, the damage it causes to DNA can be assayed using techniques such as DHPLC, LC-MS, and denaturing polyacrylamide gel electrophoresis (PAGE) .
Metabolic Pathways
It is known to interact with enzymes such as polymerase during PCR .
準備方法
The synthesis of 5-Bromo-2’-deoxycytidine typically involves the bromination of 2’-deoxycytidine. One common method includes the use of bromine or N-bromosuccinimide (NBS) in an appropriate solvent such as acetic acid or dichloromethane . The reaction conditions often require careful control of temperature and reaction time to ensure the selective bromination at the 5-position of the cytidine ring.
In industrial settings, the production of 5-Bromo-2’-deoxycytidine may involve similar bromination reactions but on a larger scale, with optimized conditions for yield and purity. The use of continuous flow reactors and automated systems can enhance the efficiency and scalability of the process .
化学反応の分析
5-Bromo-2’-deoxycytidine undergoes several types of chemical reactions, including:
Substitution Reactions: The bromine atom at the 5-position can be substituted with other nucleophiles, such as thiols or amines, under appropriate conditions.
Photochemical Reactions: When exposed to ultraviolet light, 5-Bromo-2’-deoxycytidine can induce DNA cross-linking and strand breaks.
Oxidation and Reduction: The compound can participate in redox reactions, although these are less commonly studied compared to its photochemical properties.
Common reagents used in these reactions include N-bromosuccinimide for bromination, thiols or amines for substitution, and ultraviolet light for photochemical reactions .
類似化合物との比較
5-Bromo-2’-deoxycytidine is similar to other brominated nucleosides, such as 5-bromo-2’-deoxyuridine and 8-bromo-2’-deoxyguanosine. it is unique in its specific photochemical properties and the types of DNA damage it induces .
5-Bromo-2’-deoxyuridine: Also a DNA photosensitizer, but it primarily induces different types of DNA damage compared to 5-Bromo-2’-deoxycytidine.
8-Bromo-2’-deoxyguanosine: Known for its efficient photodebromination, but it has different applications and mechanisms of action compared to 5-Bromo-2’-deoxycytidine.
生物活性
5-Bromo-2'-deoxycytidine (BrdC) is a halogenated nucleoside analog of deoxycytidine, primarily studied for its biological activity in the context of DNA incorporation, photosensitization, and potential therapeutic applications. This article provides a comprehensive overview of its biological effects, mechanisms of action, and relevant case studies.
Overview of 5-Bromo-2'-deoxycytidine
BrdC is structurally similar to deoxycytidine, with a bromine atom at the 5-position of the pyrimidine ring. It is incorporated into DNA during replication and has been shown to exhibit significant biological activity, particularly in cancer research and photodynamic therapy.
BrdC's incorporation into DNA can lead to several critical biological effects:
- DNA Damage Response : BrdC has been shown to activate DNA damage signaling pathways. In studies involving A549 lung cancer cells, BrdC induced a DNA damage response characterized by the activation of checkpoint kinases Chk1 and Chk2, as well as p53, leading to cell cycle arrest and phenotypic changes resembling senescence .
- Photosensitization : As a potential photosensitizer, BrdC can generate reactive species upon UV irradiation, leading to single-strand breaks (SSBs) and intrastrand cross-links (ICLs) in DNA. Research indicated that BrdC is less effective than its analog 5-bromo-2'-deoxyuridine (BrdU) in this capacity but still demonstrates significant photosensitizing properties .
Table 1: Summary of Biological Effects Induced by 5-Bromo-2'-deoxycytidine
Case Studies
- Lung Cancer Cells : In a study focusing on A549 lung cancer cells, BrdC exposure led to significant alterations in cell morphology and proliferation rates. The cells exhibited features typical of senescence after seven days of treatment, highlighting BrdC's impact on cellular aging processes .
- Neurogenesis Research : BrdC has been utilized as a marker for tracking neuroblast proliferation during embryonic development. Studies demonstrated that intracerebroventricular administration resulted in significantly higher labeling of neuroblasts compared to other administration routes .
- Photodynamic Therapy Applications : Research into the photosensitizing properties of BrdC revealed its potential utility in photodynamic therapy (PDT). Although less effective than BrdU, BrdC's ability to induce DNA damage under UV light suggests possible applications in treating hypoxic tumors where traditional PDT methods may fail due to oxygen dependency .
特性
IUPAC Name |
4-amino-5-bromo-1-[4-hydroxy-5-(hydroxymethyl)oxolan-2-yl]pyrimidin-2-one | |
---|---|---|
Details | Computed by Lexichem TK 2.7.0 (PubChem release 2021.05.07) | |
Source | PubChem | |
URL | https://pubchem.ncbi.nlm.nih.gov | |
Description | Data deposited in or computed by PubChem | |
InChI |
InChI=1S/C9H12BrN3O4/c10-4-2-13(9(16)12-8(4)11)7-1-5(15)6(3-14)17-7/h2,5-7,14-15H,1,3H2,(H2,11,12,16) | |
Details | Computed by InChI 1.0.6 (PubChem release 2021.05.07) | |
Source | PubChem | |
URL | https://pubchem.ncbi.nlm.nih.gov | |
Description | Data deposited in or computed by PubChem | |
InChI Key |
KISUPFXQEHWGAR-UHFFFAOYSA-N | |
Details | Computed by InChI 1.0.6 (PubChem release 2021.05.07) | |
Source | PubChem | |
URL | https://pubchem.ncbi.nlm.nih.gov | |
Description | Data deposited in or computed by PubChem | |
Canonical SMILES |
C1C(C(OC1N2C=C(C(=NC2=O)N)Br)CO)O | |
Details | Computed by OEChem 2.3.0 (PubChem release 2021.05.07) | |
Source | PubChem | |
URL | https://pubchem.ncbi.nlm.nih.gov | |
Description | Data deposited in or computed by PubChem | |
Molecular Formula |
C9H12BrN3O4 | |
Details | Computed by PubChem 2.1 (PubChem release 2021.05.07) | |
Source | PubChem | |
URL | https://pubchem.ncbi.nlm.nih.gov | |
Description | Data deposited in or computed by PubChem | |
Molecular Weight |
306.11 g/mol | |
Details | Computed by PubChem 2.1 (PubChem release 2021.05.07) | |
Source | PubChem | |
URL | https://pubchem.ncbi.nlm.nih.gov | |
Description | Data deposited in or computed by PubChem | |
CAS No. |
1022-79-3 | |
Record name | Brcdr | |
Source | DTP/NCI | |
URL | https://dtp.cancer.gov/dtpstandard/servlet/dwindex?searchtype=NSC&outputformat=html&searchlist=61765 | |
Description | The NCI Development Therapeutics Program (DTP) provides services and resources to the academic and private-sector research communities worldwide to facilitate the discovery and development of new cancer therapeutic agents. | |
Explanation | Unless otherwise indicated, all text within NCI products is free of copyright and may be reused without our permission. Credit the National Cancer Institute as the source. | |
Retrosynthesis Analysis
AI-Powered Synthesis Planning: Our tool employs the Template_relevance Pistachio, Template_relevance Bkms_metabolic, Template_relevance Pistachio_ringbreaker, Template_relevance Reaxys, Template_relevance Reaxys_biocatalysis model, leveraging a vast database of chemical reactions to predict feasible synthetic routes.
One-Step Synthesis Focus: Specifically designed for one-step synthesis, it provides concise and direct routes for your target compounds, streamlining the synthesis process.
Accurate Predictions: Utilizing the extensive PISTACHIO, BKMS_METABOLIC, PISTACHIO_RINGBREAKER, REAXYS, REAXYS_BIOCATALYSIS database, our tool offers high-accuracy predictions, reflecting the latest in chemical research and data.
Strategy Settings
Precursor scoring | Relevance Heuristic |
---|---|
Min. plausibility | 0.01 |
Model | Template_relevance |
Template Set | Pistachio/Bkms_metabolic/Pistachio_ringbreaker/Reaxys/Reaxys_biocatalysis |
Top-N result to add to graph | 6 |
Feasible Synthetic Routes
試験管内研究製品の免責事項と情報
BenchChemで提示されるすべての記事および製品情報は、情報提供を目的としています。BenchChemで購入可能な製品は、生体外研究のために特別に設計されています。生体外研究は、ラテン語の "in glass" に由来し、生物体の外で行われる実験を指します。これらの製品は医薬品または薬として分類されておらず、FDAから任何の医療状態、病気、または疾患の予防、治療、または治癒のために承認されていません。これらの製品を人間または動物に体内に導入する形態は、法律により厳格に禁止されています。これらのガイドラインに従うことは、研究と実験において法的および倫理的な基準の遵守を確実にするために重要です。