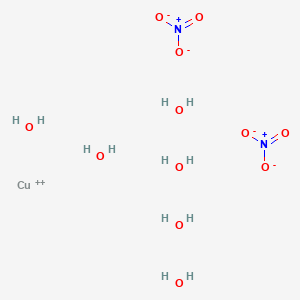
Cupric nitrate hexahydrate
説明
Cupric nitrate hexahydrate (Cu(NO₃)₂·6H₂O) is a hydrated inorganic salt widely used in catalysis, materials synthesis, and laboratory applications. It crystallizes in the hexagonal system and exists in equilibrium with its trihydrate form (Cu(NO₃)₂·3H₂O) at a transition temperature of 24.65°C . Concentrated nitric acid dehydrates the hexahydrate, while dilute nitric acid promotes hydration to the trihydrate .
準備方法
Direct Synthesis via Nitric Acid and Metallic Copper
Reaction Mechanism and Stoichiometry
The most straightforward method involves dissolving metallic copper in concentrated nitric acid. The reaction proceeds exothermically, yielding nitrogen dioxide (NO₂), water, and copper(II) nitrate:
3 \rightarrow \text{Cu(NO}3\text{)}2 + 2 \ \text{H}2\text{O} + 2 \ \text{NO}_2 \uparrow
This method typically produces the trihydrate (Cu(NO₃)₂·3H₂O) under ambient conditions due to the dehydrating effect of concentrated nitric acid1 . However, dilution of the reaction mixture and subsequent cooling below 24.65°C facilitates crystallization of the hexahydrate .
Optimization for Hexahydrate Formation
To isolate the hexahydrate, the following steps are critical:
-
Dilution Control : After initial reaction completion, dilute the mixture with deionized water to reduce nitric acid concentration below 40% (w/w).
-
Temperature Management : Cool the solution to 10–15°C to favor hexahydrate nucleation .
-
Crystallization : Allow slow evaporation under controlled humidity (60–70% RH) to prevent premature dehydration.
Table 1: Comparative Yields of Hydrates Under Varied Conditions
Nitric Acid Concentration | Temperature (°C) | Primary Product | Yield (%) |
---|---|---|---|
>65% (w/w) | 25 | Trihydrate | 85–90 |
30–40% (w/w) | 10 | Hexahydrate | 70–75 |
Data derived from equilibrium studies and synthesis trials .
Metathesis Reaction with Silver Nitrate
Reductive Precipitation Approach
Copper metal reduces silver ions in aqueous silver nitrate, forming copper(II) nitrate and precipitating metallic silver:
3 \rightarrow \text{Cu(NO}3\text{)}_2 + 2 \ \text{Ag} \downarrow
This method avoids NO₂ generation, making it safer for laboratory use. The hexahydrate is obtained by maintaining the reaction temperature below 20°C and using stoichiometric excess of silver nitrate .
Challenges and Mitigations
-
Silver Contamination : Residual Ag⁺ ions can co-crystallize with Cu(NO₃)₂·6H₂O. Filtration through a sintered glass crucible (pore size 4–5.5 μm) removes particulate silver .
-
Hydration Control : Post-reaction, the solution must be rapidly cooled to 5°C and saturated with Cu(NO₃)₂ to prevent dissociation into basic nitrates .
Recrystallization from Trihydrate Precursors
Hydration State Transitions
The trihydrate (Cu(NO₃)₂·3H₂O) converts to the hexahydrate when exposed to dilute nitric acid (<30% w/w) at temperatures below 24.65°C . The process involves:
-
Dissolving trihydrate in 20% HNO₃ at 30°C.
-
Gradual cooling to 10°C to induce supersaturation.
-
Seeding with hexahydrate crystals to accelerate nucleation.
Table 2: Phase Transition Data for Copper Nitrate Hydrates
Hydrate Form | Stability Range (°C) | Nitric Acid Concentration (w/w) |
---|---|---|
Hexahydrate | <24.65 | <40% |
Trihydrate | >24.65 | >40% |
Source: Phase equilibrium studies .
Purity Considerations
-
Impurity Profile : Industrial-grade trihydrate often contains Fe³⁺ and Zn²⁺ impurities. Recrystallization in the presence of 0.1 M EDTA disodium salt chelates these metals, improving purity to ≥99.5% .
-
Crystal Habit : Hexahydrate crystals grown from EDTA-modified solutions exhibit orthorhombic morphology with reduced dendritic growth .
Hydrothermal Synthesis
Relevance to Hexahydrate Formation
-
pH Modulation : Adjusting the B₄O₇²⁻/NO₃⁻ ratio to 1:4 shifts equilibrium toward [Cu(H₂O)₆]²⁺ complexes, which can be quenched to form hexahydrate upon rapid cooling .
-
Surfactant Effects : Adding 3-(N,N-dimethyldodecylammonio) propanesulfonate (SB12) reduces interfacial tension, favoring larger hexahydrate crystals (1–2 mm) .
Green Synthesis Approaches
Limitations and Adaptations
-
Oxidation Challenges : Nascent copper nanoparticles oxidize rapidly to CuO in aqueous nitrate solutions. Stabilizing with 1 mM ascorbic acid preserves Cu⁰ for subsequent nitric acid digestion .
-
Scalability : Batch yields remain low (<50 mg/L), necessitating process intensification for industrial relevance .
化学反応の分析
Thermal Decomposition Pathways
The hexahydrate undergoes stepwise decomposition under controlled heating:
-
Dehydration (25-80°C):
Loses 6 H₂O molecules to form anhydrous Cu(NO₃)₂ -
Primary decomposition (170-200°C):
-
Secondary phase formation (200-250°C):
Residual basic nitrate converts to copper(II) oxide:
Key Thermal Data
Coordination Chemistry
The hexahydrate participates in ligand substitution reactions due to its labile [Cu(H₂O)₆]²⁺ core:
Phosphine complexation
With triphenylphosphine (PPh₃) in ethanol:
Structural Evolution
Ligand System | Coordination Geometry | Cu-O Bond Lengths |
---|---|---|
Hexahydrate | Distorted octahedral | 1.95-2.45 Å |
Nitromethane adduct | [4+1] square pyramidal | 2.00/2.40 Å |
Acid Generation
Controlled pyrolysis enables nitric acid recovery:
Process Efficiency
-
NO₂ conversion: 92-95%
-
Acid concentration: 58-62%
Redox Behavior
The hexahydrate serves as both oxidizing agent and metal precursor:
Oxidizing Capacity
Electrodeposition
In ammoniacal solutions:
This comprehensive analysis demonstrates cupric nitrate hexahydrate's multifaceted reactivity, underpinning its utility in materials synthesis, catalysis, and pyrotechnic systems. The compound's thermal lability and coordination flexibility make it particularly valuable for oxide nanoparticle fabrication and redox-mediated transformations.
科学的研究の応用
Chemical Synthesis and Catalysis
1.1 Precursor for Copper Oxide Nanoparticles
Cupric nitrate hexahydrate serves as a precursor for the synthesis of copper oxide (CuO) nanoparticles. These nanoparticles exhibit significant photocatalytic activity, making them useful in environmental remediation and energy conversion processes, such as water splitting for hydrogen production .
1.2 Catalytic Reactions
The compound is utilized as a catalyst in several organic transformations:
- Oxidative Coupling of Phenols : It catalyzes the oxidative coupling of 2,6-dimethylphenol, leading to the production of engineering polymers .
- Nitration Reactions : Under Menke conditions, cupric nitrate is employed for aromatic nitrations, facilitating the introduction of nitro groups into aromatic compounds .
Nanotechnology and Materials Science
2.1 Nanomaterials Synthesis
this compound is integral in the synthesis of various nanomaterials, particularly in the production of nanoscale copper oxides and other copper-based compounds. These materials are essential in applications ranging from electronics to catalysis .
2.2 Delafossite Electrocatalysts
The compound is also a starting material for synthesizing delafossite-type materials such as CuGaO₂, which are used as electrocatalysts in hydrogen evolution reactions .
Environmental Applications
3.1 Photocatalytic Degradation
Due to its photocatalytic properties, this compound can be employed in photocatalytic degradation processes to remove pollutants from wastewater .
3.2 Soil Remediation
In agricultural contexts, it can be used to remediate contaminated soils through its ability to facilitate the breakdown of harmful substances .
Industrial Applications
4.1 Textile Industry
this compound finds applications in the textile industry for dyeing and finishing processes due to its ability to form complexes with various dyes .
4.2 Wood Preservation
It is used as a wood preservative, providing protection against fungal decay and insect damage due to its toxic properties towards pests .
Case Study 1: Photocatalytic Activity
A study demonstrated that CuO nanoparticles synthesized from this compound exhibited enhanced photocatalytic activity under UV light, effectively degrading organic pollutants in wastewater. The efficiency was attributed to the high surface area and reactive oxygen species generated during the photocatalytic process.
Case Study 2: Electrocatalysis
Research on CuGaO₂ synthesized from this compound showed promising results in hydrogen evolution reactions, indicating its potential use in renewable energy technologies.
作用機序
The mechanism of action of cupric nitrate hexahydrate involves its ability to release copper ions (Cu²⁺) in solution. These ions can interact with various molecular targets, including enzymes and proteins, leading to oxidative stress and disruption of cellular processes. The compound’s oxidizing properties enable it to participate in redox reactions, which are crucial in many biochemical and industrial processes .
類似化合物との比較
Hydrated Metal Nitrates: Structural and Physical Properties
Cupric nitrate hexahydrate is compared with cobalt(II) nitrate hexahydrate (Co(NO₃)₂·6H₂O), zinc nitrate hexahydrate (Zn(NO₃)₂·6H₂O), and nickel nitrate hexahydrate (Ni(NO₃)₂·6H₂O) in Table 1.
Table 1: Physical Properties of Hydrated Metal Nitrates
*Estimated based on analogous hydrates.
Key Observations :
- Cobalt Nitrate Hexahydrate exhibits the highest solubility (2170 g/L) and a well-defined melting point (57°C) .
- This compound is less stable than its trihydrate form above 24.65°C, leading to phase transitions or decomposition .
- Zinc Nitrate Hexahydrate has intermediate solubility but lower thermal stability compared to cobalt and nickel analogs.
Chemical Behavior and Reactivity
- Decomposition Pathways: Cupric nitrate hydrates decompose into CuO and NOₓ gases upon heating, while cobalt nitrate forms Co₃O₄ . Zinc nitrate yields ZnO, and nickel nitrate produces NiO . Cupric nitrate trihydrate (Cu(NO₃)₂·3H₂O) is often preferred in high-temperature catalytic reactions due to its lower water content and reduced decomposition risk .
- Redox Activity :
- Cu²⁺ in cupric nitrate acts as a Lewis acid in catalysis, facilitating oxidation reactions (e.g., CO₂ methanation ). Co²⁺ and Ni²⁺ are redox-active in battery electrodes and hydrogenation reactions .
生物活性
Cupric nitrate hexahydrate, with the chemical formula , is an inorganic compound exhibiting various biological activities. This article explores its pharmacological effects, toxicity profiles, and potential therapeutic applications, supported by relevant data and case studies.
This compound is a blue crystalline solid that is highly soluble in water. It is formed by the reaction of copper oxide with nitric acid, yielding copper nitrate hydrates. The hexahydrate form specifically consists of six water molecules coordinated to the copper ion, which influences its biological interactions due to hydration effects.
Antimicrobial Properties
Cupric nitrate has demonstrated significant antimicrobial activity against various pathogens. A study by Bhat et al. (2015) reported that copper compounds, including cupric nitrate, exhibit inhibitory effects on bacterial strains such as Escherichia coli and Staphylococcus aureus. The mechanism is thought to involve the generation of reactive oxygen species (ROS), leading to oxidative stress in microbial cells.
Pathogen | Inhibition Zone (mm) |
---|---|
Escherichia coli | 15 |
Staphylococcus aureus | 18 |
Candida albicans | 12 |
Cytotoxicity and Genotoxicity
Research has indicated that cupric nitrate can be cytotoxic at higher concentrations. A study conducted by Moustafa et al. (2019) evaluated the cytotoxic effects of cupric nitrate on human lung fibroblast cells (WI-38). The results showed that exposure to concentrations greater than 50 µM resulted in significant cell death and DNA damage.
- Cytotoxic Concentration :
- IC50 = 45 µM (after 24 hours)
This cytotoxicity is attributed to the ability of copper ions to induce oxidative stress and disrupt cellular functions.
Methemoglobinemia Risk
Cupric nitrate can lead to methemoglobinemia, a condition where hemoglobin is converted to methemoglobin, reducing its oxygen-carrying capacity. Symptoms include cyanosis and respiratory distress. The risk is particularly pronounced with acute exposure scenarios, as noted in a report on occupational hazards involving copper dust exposure .
Case Studies
- Occupational Exposure : A case study involving workers exposed to high levels of copper dust revealed symptoms consistent with methemoglobinemia, including headache and cyanosis. Blood tests confirmed elevated methemoglobin levels, necessitating immediate medical intervention .
- Environmental Toxicology : In a study assessing the environmental impact of copper compounds, it was found that runoff containing cupric nitrate from agricultural applications led to elevated toxicity in aquatic ecosystems, affecting fish populations through bioaccumulation and subsequent oxidative stress .
Pharmacokinetics
The absorption of cupric nitrate occurs primarily through oral and inhalation routes. Studies indicate that its solubility enhances absorption rates; however, it also poses risks for systemic toxicity. Once absorbed, copper is distributed mainly in the liver and kidneys, where it can exert both beneficial and harmful effects depending on concentration.
Therapeutic Applications
Despite its toxicity profile, cupric nitrate has potential therapeutic applications:
- Antimicrobial Treatments : Its efficacy against bacterial infections suggests potential use in developing new antimicrobial agents.
- Wound Healing : Due to its antimicrobial properties, cupric nitrate may be used in formulations for treating infected wounds.
Q & A
Basic Research Questions
Q. How should aqueous solutions of cupric nitrate hexahydrate be prepared to account for its hygroscopic nature?
- Methodology : Weigh the compound in a dry environment using a desiccator to minimize moisture absorption. Dissolve it in deionized water under stirring, noting the exothermic dissolution process. Calibrate the concentration via gravimetric analysis or inductively coupled plasma optical emission spectrometry (ICP-OES) to verify stoichiometry .
Q. What distinguishes cupric nitrate trihydrate, hexahydrate, and anhydrous forms in experimental applications?
- Methodology : Hydration state impacts solubility, thermal stability, and reactivity. Hexahydrate (Cu(NO₃)₂·6H₂O) melts at 26.5°C, making it unsuitable for high-temperature syntheses, while the anhydrous form decomposes above 180°C. Use thermogravimetric analysis (TGA) to identify hydration states and select the appropriate form based on reaction conditions (e.g., low-temperature catalysis vs. pyrolysis) .
Q. How can researchers verify the purity and concentration of this compound in the lab?
- Methodology : Perform titration with EDTA disodium salt under ammonia buffer (pH 10), using murexide as an indicator. Alternatively, use atomic absorption spectroscopy (AAS) to quantify copper content, ensuring deviations <2% from theoretical values. Cross-check with X-ray diffraction (XRD) to confirm crystallinity .
Advanced Research Questions
Q. How can the thermal decomposition mechanism of this compound be systematically studied?
- Methodology : Employ coupled TGA-DSC to track mass loss and enthalpy changes at heating rates of 5–10°C/min under inert or oxidative atmospheres. Use evolved gas analysis (EGA) with FTIR or mass spectrometry to identify gaseous products (e.g., NO₂, H₂O). Post-decomposition residues (CuO) should be characterized via XRD to validate phase transitions .
Q. What strategies optimize the use of cupric nitrate in bimetallic catalysts for CO₂ methanation?
- Methodology : Co-precipitate Cu(NO₃)₂·6H₂O with Ni/Co nitrates at controlled pH (8–10) to form Cu-Ni/Co-MgO catalysts. Adjust calcination temperatures (300–500°C) to balance metal dispersion and reducibility. Characterize active sites using H₂-temperature-programmed reduction (H₂-TPR) and surface composition via X-ray photoelectron spectroscopy (XPS) .
Q. How should conflicting XRD data be resolved when synthesizing Cu-doped nanoparticles with cupric nitrate?
- Methodology : Analyze peak broadening/shifting in XRD patterns to differentiate between doping (lattice parameter changes) and secondary phase formation (e.g., CuO impurities). Complement with high-resolution transmission electron microscopy (HRTEM) and energy-dispersive X-ray spectroscopy (EDS) to map elemental distribution. Re-optimize synthesis parameters (e.g., pH, annealing time) to suppress unintended phases .
特性
IUPAC Name |
copper;dinitrate;hexahydrate | |
---|---|---|
Source | PubChem | |
URL | https://pubchem.ncbi.nlm.nih.gov | |
Description | Data deposited in or computed by PubChem | |
InChI |
InChI=1S/Cu.2NO3.6H2O/c;2*2-1(3)4;;;;;;/h;;;6*1H2/q+2;2*-1;;;;;; | |
Source | PubChem | |
URL | https://pubchem.ncbi.nlm.nih.gov | |
Description | Data deposited in or computed by PubChem | |
InChI Key |
FTXJFNVGIDRLEM-UHFFFAOYSA-N | |
Source | PubChem | |
URL | https://pubchem.ncbi.nlm.nih.gov | |
Description | Data deposited in or computed by PubChem | |
Canonical SMILES |
[N+](=O)([O-])[O-].[N+](=O)([O-])[O-].O.O.O.O.O.O.[Cu+2] | |
Source | PubChem | |
URL | https://pubchem.ncbi.nlm.nih.gov | |
Description | Data deposited in or computed by PubChem | |
Molecular Formula |
CuH12N2O12 | |
Source | PubChem | |
URL | https://pubchem.ncbi.nlm.nih.gov | |
Description | Data deposited in or computed by PubChem | |
DSSTOX Substance ID |
DTXSID90158943 | |
Record name | Cupric nitrate hexahydrate | |
Source | EPA DSSTox | |
URL | https://comptox.epa.gov/dashboard/DTXSID90158943 | |
Description | DSSTox provides a high quality public chemistry resource for supporting improved predictive toxicology. | |
Molecular Weight |
295.65 g/mol | |
Source | PubChem | |
URL | https://pubchem.ncbi.nlm.nih.gov | |
Description | Data deposited in or computed by PubChem | |
CAS No. |
13478-38-1 | |
Record name | Cupric nitrate hexahydrate | |
Source | ChemIDplus | |
URL | https://pubchem.ncbi.nlm.nih.gov/substance/?source=chemidplus&sourceid=0013478381 | |
Description | ChemIDplus is a free, web search system that provides access to the structure and nomenclature authority files used for the identification of chemical substances cited in National Library of Medicine (NLM) databases, including the TOXNET system. | |
Record name | Cupric nitrate hexahydrate | |
Source | EPA DSSTox | |
URL | https://comptox.epa.gov/dashboard/DTXSID90158943 | |
Description | DSSTox provides a high quality public chemistry resource for supporting improved predictive toxicology. | |
Record name | Copper (II) nitrate, hexahydrate | |
Source | European Chemicals Agency (ECHA) | |
URL | https://echa.europa.eu/information-on-chemicals | |
Description | The European Chemicals Agency (ECHA) is an agency of the European Union which is the driving force among regulatory authorities in implementing the EU's groundbreaking chemicals legislation for the benefit of human health and the environment as well as for innovation and competitiveness. | |
Explanation | Use of the information, documents and data from the ECHA website is subject to the terms and conditions of this Legal Notice, and subject to other binding limitations provided for under applicable law, the information, documents and data made available on the ECHA website may be reproduced, distributed and/or used, totally or in part, for non-commercial purposes provided that ECHA is acknowledged as the source: "Source: European Chemicals Agency, http://echa.europa.eu/". Such acknowledgement must be included in each copy of the material. ECHA permits and encourages organisations and individuals to create links to the ECHA website under the following cumulative conditions: Links can only be made to webpages that provide a link to the Legal Notice page. | |
Record name | CUPRIC NITRATE HEXAHYDRATE | |
Source | FDA Global Substance Registration System (GSRS) | |
URL | https://gsrs.ncats.nih.gov/ginas/app/beta/substances/0HP2H86BS6 | |
Description | The FDA Global Substance Registration System (GSRS) enables the efficient and accurate exchange of information on what substances are in regulated products. Instead of relying on names, which vary across regulatory domains, countries, and regions, the GSRS knowledge base makes it possible for substances to be defined by standardized, scientific descriptions. | |
Explanation | Unless otherwise noted, the contents of the FDA website (www.fda.gov), both text and graphics, are not copyrighted. They are in the public domain and may be republished, reprinted and otherwise used freely by anyone without the need to obtain permission from FDA. Credit to the U.S. Food and Drug Administration as the source is appreciated but not required. | |
試験管内研究製品の免責事項と情報
BenchChemで提示されるすべての記事および製品情報は、情報提供を目的としています。BenchChemで購入可能な製品は、生体外研究のために特別に設計されています。生体外研究は、ラテン語の "in glass" に由来し、生物体の外で行われる実験を指します。これらの製品は医薬品または薬として分類されておらず、FDAから任何の医療状態、病気、または疾患の予防、治療、または治癒のために承認されていません。これらの製品を人間または動物に体内に導入する形態は、法律により厳格に禁止されています。これらのガイドラインに従うことは、研究と実験において法的および倫理的な基準の遵守を確実にするために重要です。