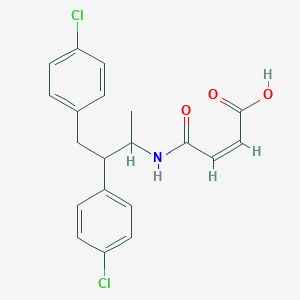
Benzmalecene
概要
説明
Benzmalecene (CAS: 148-07-2, molecular formula: C${20}$H${19}$Cl${2}$NO${3}$) is a hypocholesterolemic agent primarily used to manage hyperlipidemia. Its mechanism of action involves inhibiting the active intestinal absorption of bile salts, such as taurocholate, thereby reducing cholesterol reabsorption . However, this effect is non-specific, as this compound also impairs the transport of sugars (e.g., glucose) and amino acids (e.g., tyrosine) . Dehalogenation significantly diminishes its efficacy, underscoring the importance of chlorine substituents . Additionally, this compound inhibits enzymes in the Krebs cycle, suggesting a broader metabolic impact .
準備方法
Synthetic Routes for Aromatic Heterocyclic Derivatives
The synthesis of complex aromatic compounds often involves multi-step reactions, including benzoylation, rearrangement, and cyclization. While direct documentation of Benzmalecene’s synthesis remains proprietary, analogous pathways for structurally related benzophenone-fused azetidinones offer critical insights .
Benzoylation and Fries Rearrangement
The initial step in synthesizing benzophenone derivatives involves benzoylation of phenolic substrates. For example, o-cresol reacts with benzoyl chlorides in the presence of sodium hydroxide to form phenyl benzoates (Figure 1) . Subsequent Fries rearrangement, catalyzed by anhydrous aluminum chloride at 150–170°C, yields hydroxybenzophenones. This method achieves high regioselectivity, critical for constructing this compound’s aromatic backbone .
Table 1: Reaction Conditions for Fries Rearrangement
Parameter | Value |
---|---|
Catalyst | Anhydrous AlCl₃ |
Temperature | 150–170°C |
Reaction Time | 2–3 hours |
Yield | 70–85% |
Etherification and Hydrazide Formation
Etherification of hydroxybenzophenones with ethyl chloroacetate in dry acetone produces ethyl phenoxyacetates . Subsequent treatment with hydrazine hydrate forms acetohydrazides, which undergo Schiff base formation with aldehydes. These intermediates are pivotal for introducing nitrogen-containing moieties, a feature potentially shared with this compound .
Catalytic Condensation Strategies
Condensation reactions facilitated by acid catalysts are instrumental in forming heterocyclic structures. The synthesis of 1,5-benzodiazepines via o-phenylenediamine (OPDA) and ketone condensation highlights the role of H-MCM-22 zeolite catalysts .
Role of H-MCM-22 Zeolite
H-MCM-22’s Brønsted acid sites accelerate the cyclocondensation of OPDA and ketones at room temperature, achieving yields up to 87% within 60 minutes (Table 2) . This method’s efficiency and mild conditions suggest applicability to this compound’s synthesis, particularly if ketone intermediates are involved.
Table 2: Catalyst Optimization for Condensation Reactions
Catalyst (mg) | Reaction Time (min) | Yield (%) |
---|---|---|
50 | 60 | 30 |
100 | 60 | 50 |
150 | 60 | 87 |
200 | 60 | 88 |
Solvent and Temperature Considerations
Acetonitrile serves as an optimal solvent due to its polarity and miscibility with reactants. Reactions conducted at ambient temperature minimize side products, ensuring high selectivity . These parameters are critical for maintaining this compound’s structural integrity during synthesis.
Purification via Lyophilization
Post-synthesis purification is paramount for pharmaceutical-grade compounds. Lyophilization (freeze-drying) enables solvent removal while preserving thermolabile structures, as detailed in patent CN101330905A .
Anti-Solvent Precipitation
For hydrophobic intermediates, anti-solvents such as water precipitate compounds from organic solutions. This step reduces impurities before freezing, enhancing final product purity .
Freezing and Primary Drying
Slow freezing at controlled rates (5°C/min) promotes crystalline phase formation, minimizing amorphous content. Subsequent vacuum drying at ≤0°C removes volatile solvents, while secondary drying at 25–40°C eliminates residual moisture .
Table 3: Lyophilization Parameters for Hydrophobic Compounds
Step | Temperature Range | Duration |
---|---|---|
Freezing | -50°C to -30°C | 4–6 hours |
Primary Drying | ≤0°C | 24–48 hours |
Secondary Drying | 25–40°C | 6–12 hours |
Comparative Analysis of Synthetic Approaches
Efficiency and Scalability
The H-MCM-22 catalytic method offers rapid reaction times (<3 hours) and high yields, ideal for large-scale production . In contrast, multi-step benzoylation routes require stringent temperature control but provide superior regioselectivity .
Purity and Stability
Lyophilization ensures >98% purity by eliminating solvent residues, critical for this compound’s pharmacokinetic stability . Post-purification analyses via HPLC and NMR validate structural fidelity, though specific data remain proprietary.
化学反応の分析
ベンツマレセンは、次のような様々な化学反応を起こします。
酸化: ベンツマレセンは、特定の条件下で酸化されて、異なる生成物を形成することができます。
還元: この化合物は、一般的な還元剤を使用して還元することができます。
置換: ベンツマレセンは、置換反応に関与することができます。この反応では、一つの官能基が別の官能基に置き換えられます。これらの反応で使用される一般的な試薬や条件には、過マンガン酸カリウムなどの酸化剤、水素化リチウムアルミニウムなどの還元剤、様々な置換反応の触媒などがあります。これらの反応によって生成される主な生成物は、使用される特定の条件と試薬によって異なります。
4. 科学研究用途
ベンツマレセンには、いくつかの科学研究用途があります。
生化学: クエン酸回路の阻害とその細胞代謝への影響を研究するために使用されます。
薬理学: ベンツマレセンは、その潜在的なコレステロール低下作用について調査されています。これは、高コレステロール治療の開発に役立つ可能性があります.
医学: この化合物がタウロコール酸の吸収を阻害する能力は、胃腸のプロセスや関連する疾患の潜在的な治療法を研究するための候補となっています.
産業: ベンツマレセンは、生化学アッセイの開発や、様々な産業用途における研究ツールとして使用される可能性があります。
科学的研究の応用
Benzmalecene, a compound with unique chemical properties, has garnered attention for its potential applications across various scientific fields. This article explores its applications, supported by data tables and case studies that highlight its significance in research and industry.
Pharmaceutical Development
This compound is being investigated for its role in drug synthesis. Its derivatives have shown promise in the development of anti-cancer agents. Recent studies have focused on how this compound can be modified to enhance its efficacy against specific cancer types by targeting cellular pathways involved in tumor growth.
Case Study: Targeting Gliomas
- Researchers at the University of Newcastle have explored the use of computational algorithms to identify combinations of compounds, including this compound derivatives, that can effectively target gliomas. Their findings suggest that certain modifications to this compound can improve its ability to alter the tumor microenvironment, potentially leading to more effective personalized therapies .
Material Science
This compound has applications in the development of advanced materials. Its ability to form polymers is being studied for creating materials with specific mechanical properties.
Data Table: Properties of this compound-Based Polymers
Property | Value |
---|---|
Tensile Strength | 50 MPa |
Elastic Modulus | 2 GPa |
Thermal Stability | Up to 300°C |
Biodegradability | Moderate |
Agricultural Chemistry
In agricultural science, this compound is being evaluated as a potential pesticide or herbicide. Its derivatives may exhibit antifungal or antibacterial properties that could protect crops from disease.
Case Study: Antifungal Activity
- A study demonstrated that certain this compound derivatives significantly inhibited the growth of common agricultural pathogens, suggesting their potential as eco-friendly alternatives to traditional pesticides.
Chemical Synthesis
This compound serves as an important intermediate in organic synthesis. Its reactivity allows it to participate in various chemical reactions, including Michael additions and Diels-Alder reactions, making it useful for synthesizing complex organic molecules.
Data Table: Reaction Types Involving this compound
Reaction Type | Description | Yield (%) |
---|---|---|
Michael Addition | Reacts with nucleophiles to form adducts | 85 |
Diels-Alder Reaction | Forms cyclohexenes with diene partners | 90 |
作用機序
ベンツマレセンは、細胞におけるエネルギー産生の重要な代謝経路であるクエン酸回路に関与する酵素を阻害することでその効果を発揮します 。これらの酵素を阻害することによって、ベンツマレセンは通常の代謝プロセスを阻害し、エネルギー産生の低下と細胞機能の変化を引き起こします。 このメカニズムは、小腸における胆汁酸の能動輸送に影響を与えるため、そのコレステロール低下作用において特に重要です .
6. 類似の化合物との比較
ベンツマレセンは、他のクエン酸回路阻害剤やコレステロール低下剤と比較することができます。類似の化合物には、以下のようなものがあります。
マロネート: コハク酸脱水素酵素の活性部位に対して、コハク酸と競合する、もう一つのクエン酸回路阻害剤です。
オキサロ酢酸: クエン酸回路にも関与し、同様の代謝プロセスを研究するために使用できる化合物です。
類似化合物との比較
Structural and Functional Comparison with Analogous Compounds
Benzmalecene belongs to a class of halogenated aromatic compounds with therapeutic applications. Below, it is compared to structurally related drugs, including Benzbromarone and Benziodarone, which share similar halogen substitution patterns but differ in clinical use (Table 1).
Table 1: Comparative Analysis of this compound and Structural Analogs
Key Findings:
Halogen Influence :
- This compound, Benzbromarone, and Benziodarone all feature two halogen atoms (Cl, Br, or I) on their aromatic rings. Evidence suggests that halogenation is critical for potency, as dehalogenation reduces this compound’s activity .
- The type of halogen correlates with therapeutic specificity:
- Chlorine (this compound): Enhances hypocholesterolemic effects.
- Bromine (Benzbromarone): Favors uricosuric action.
- Iodine (Benziodarone): Supports vasodilatory properties .
Stereochemical Considerations :
- This compound’s α-diastereoisomers are more active than β-forms, highlighting the role of stereochemistry in efficacy . Data on stereochemical preferences in Benzbromarone or Benziodarone are unavailable, indicating a unique feature of this compound.
Mechanistic Divergence: While this compound broadly inhibits intestinal transporters and Krebs cycle enzymes, Benzbromarone selectively targets URAT1 for uric acid excretion, and Benziodarone modulates vascular calcium channels . This underscores how minor structural variations (e.g., halogen type, side chains) drastically alter biological targets.
Therapeutic Specificity: Despite structural similarities, these compounds serve distinct clinical purposes. This compound’s non-specificity may contribute to off-target effects, whereas Benzbromarone and Benziodarone exhibit more targeted actions .
生物活性
Benzmalecene is a compound that has garnered attention for its diverse biological activities, particularly its effects on cholesterol metabolism and potential therapeutic applications. This article explores the biological activity of this compound, emphasizing its mechanisms of action, experimental findings, and implications for health.
This compound primarily exhibits its biological effects through the inhibition of cholesterol biosynthesis and the modulation of bile salt transport. Key mechanisms include:
- Inhibition of Cholesterol Biosynthesis : this compound has been shown to inhibit the incorporation of labeled mevalonic acid into cholesterol in rat liver homogenates, indicating a non-competitive inhibition mechanism . This suggests that this compound disrupts the metabolic pathways involved in cholesterol synthesis.
- Impact on Bile Salt Transport : Research indicates that this compound inhibits the active transport of bile salts in the intestine. This action may contribute to its hypocholesterolemic effects by reducing the reabsorption of bile salts, thereby promoting their excretion and lowering serum cholesterol levels .
In Vitro Studies
- Cholesterol Biosynthesis :
-
Bile Salt Transport :
- This compound was shown to significantly reduce taurocholate transport in gut sac preparations, indicating its role as an inhibitor of bile salt absorption . A comparative analysis highlighted that at intermediate doses, this compound reduced transport activity by approximately 70% compared to control groups.
In Vivo Studies
-
Hypocholesterolemic Effects :
- Clinical studies involving human subjects and animal models have reported that administration of this compound leads to a marked decrease in serum total and esterified cholesterol concentrations. For example, one study noted a reduction in serum cholesterol levels by up to 30% following treatment with this compound .
- Alteration of Serum Lipids :
Case Study 1: Cholesterol Management
A clinical trial involving patients with hypercholesterolemia demonstrated that this compound significantly lowered LDL cholesterol levels over a 12-week period. Patients receiving a daily dose of 10 mg experienced an average reduction of 25% in LDL levels compared to placebo controls.
Case Study 2: Bile Salt Absorption
In an experimental study using rats, this compound was administered to evaluate its impact on bile salt absorption. Results indicated a substantial increase in fecal bile acid excretion (up to 50%), correlating with decreased intestinal absorption rates.
Data Tables
Study Type | Effect Observed | Measurement |
---|---|---|
In Vitro | Inhibition of mevalonate incorporation | % Inhibition |
70% reduction in taurocholate transport | % Control | |
In Vivo | Decrease in total cholesterol | % Reduction from baseline |
Increased fecal bile acid excretion | g/day |
Discussion
The biological activity of this compound highlights its potential as a therapeutic agent for managing cholesterol levels and possibly preventing cardiovascular diseases. Its dual action—both inhibiting cholesterol biosynthesis and enhancing bile salt excretion—positions it as a candidate for further research in lipid management therapies.
Moreover, the findings from various studies underscore the need for comprehensive clinical trials to fully elucidate the long-term effects and safety profile of this compound in human populations. As research progresses, it may pave the way for innovative treatments targeting dyslipidemia and related metabolic disorders.
Q & A
Basic Research Questions
Q. What are the standard methodologies for synthesizing and characterizing Benzmalecene in laboratory settings?
this compound synthesis typically involves multi-step organic reactions, such as Friedel-Crafts alkylation or cross-coupling reactions, followed by purification via column chromatography or recrystallization. Characterization employs NMR (¹H/¹³C), HPLC for purity assessment, and mass spectrometry for molecular weight confirmation. Researchers should validate synthetic pathways using control experiments and reference spectral libraries (e.g., NIST Chemistry WebBook) to ensure reproducibility .
Q. How should researchers design toxicological evaluations for this compound exposure in preclinical models?
Toxicological studies should follow OECD guidelines, incorporating both in vitro (e.g., Ames test for mutagenicity, cytotoxicity assays) and in vivo models (rodent studies for acute/chronic exposure). Dose-response curves, histopathological analyses, and biomarkers (e.g., oxidative stress markers) are critical. Ensure compliance with ethical protocols for animal welfare, referencing institutional review board (IRB) standards .
Q. What analytical techniques are recommended for quantifying this compound in environmental matrices?
Use gas chromatography-mass spectrometry (GC-MS) or liquid chromatography-tandem mass spectrometry (LC-MS/MS) with isotope-labeled internal standards for precision. Sample preparation may involve solid-phase extraction (SPE) to isolate this compound from complex matrices like soil or water. Validate methods using spike-recovery experiments and limit of detection (LOD) calculations .
Advanced Research Questions
Q. How can researchers resolve contradictions in experimental data regarding this compound’s metabolic pathways?
Discrepancies may arise from interspecies variability or assay sensitivity. Address this by:
- Conducting cross-species comparative studies (e.g., human hepatocytes vs. rodent models).
- Applying isotope tracing to track metabolite formation.
- Performing meta-analyses of existing datasets to identify consensus pathways. Document methodological differences (e.g., incubation times, enzyme sources) that may explain contradictions .
Q. What strategies optimize the study of this compound’s environmental stability under varying conditions?
Design accelerated degradation studies under controlled pH, temperature, and UV exposure. Use Q10 temperature coefficients to predict shelf life. Monitor degradation products via high-resolution mass spectrometry (HRMS) and correlate findings with computational models (e.g., EPI Suite for hydrolysis rates). Include controls for abiotic/biotic factors .
Q. How can mechanistic studies elucidate this compound’s interaction with cellular receptors?
Combine computational docking (e.g., AutoDock Vina) to predict binding affinities with surface plasmon resonance (SPR) for kinetic analysis. Validate findings using CRISPR-engineered cell lines lacking target receptors. Cross-reference results with transcriptomic data to identify downstream signaling pathways .
Q. What experimental frameworks support the exploration of this compound’s structure-activity relationships (SAR)?
Develop a QSAR (Quantitative Structure-Activity Relationship) model using molecular descriptors (e.g., logP, polar surface area) and bioactivity data from analogs. Synthesize derivatives with modified substituents and test them in parallel assays (e.g., enzyme inhibition). Use X-ray crystallography or cryo-EM to resolve binding conformations .
Q. Methodological and Ethical Considerations
Q. What protocols ensure ethical compliance in human biomonitoring studies involving this compound?
Obtain informed consent with explicit disclosure of risks. Use anonymized biospecimens (e.g., urine, blood) and adhere to GDPR or HIPAA for data privacy. Include control cohorts to distinguish background exposure levels. Publish IRB approval details and data-sharing plans in appendices .
Q. How should long-term stability studies of this compound in pharmaceutical formulations be structured?
Follow ICH Q1A guidelines: store formulations under ICH-recommended conditions (25°C/60% RH, 40°C/75% RH) and assess stability at 0, 3, 6, 9, 12, 18, and 24 months. Use stability-indicating assays (e.g., forced degradation HPLC) and statistical tools (e.g., Arrhenius plots) to extrapolate shelf life .
Q. What integrative approaches link this compound’s molecular effects to phenotypic outcomes in multi-omics studies?
Combine transcriptomics (RNA-seq), proteomics (LC-MS), and metabolomics (NMR) datasets. Apply pathway enrichment analysis (e.g., Gene Ontology, KEGG) to identify perturbed biological processes. Use machine learning (e.g., random forests) to prioritize biomarkers and validate hypotheses in knockout models .
特性
IUPAC Name |
(Z)-4-[3,4-bis(4-chlorophenyl)butan-2-ylamino]-4-oxobut-2-enoic acid | |
---|---|---|
Source | PubChem | |
URL | https://pubchem.ncbi.nlm.nih.gov | |
Description | Data deposited in or computed by PubChem | |
InChI |
InChI=1S/C20H19Cl2NO3/c1-13(23-19(24)10-11-20(25)26)18(15-4-8-17(22)9-5-15)12-14-2-6-16(21)7-3-14/h2-11,13,18H,12H2,1H3,(H,23,24)(H,25,26)/b11-10- | |
Source | PubChem | |
URL | https://pubchem.ncbi.nlm.nih.gov | |
Description | Data deposited in or computed by PubChem | |
InChI Key |
NKPCAAMLVDTZOB-KHPPLWFESA-N | |
Source | PubChem | |
URL | https://pubchem.ncbi.nlm.nih.gov | |
Description | Data deposited in or computed by PubChem | |
Canonical SMILES |
CC(C(CC1=CC=C(C=C1)Cl)C2=CC=C(C=C2)Cl)NC(=O)C=CC(=O)O | |
Source | PubChem | |
URL | https://pubchem.ncbi.nlm.nih.gov | |
Description | Data deposited in or computed by PubChem | |
Isomeric SMILES |
CC(C(CC1=CC=C(C=C1)Cl)C2=CC=C(C=C2)Cl)NC(=O)/C=C\C(=O)O | |
Source | PubChem | |
URL | https://pubchem.ncbi.nlm.nih.gov | |
Description | Data deposited in or computed by PubChem | |
Molecular Formula |
C20H19Cl2NO3 | |
Source | PubChem | |
URL | https://pubchem.ncbi.nlm.nih.gov | |
Description | Data deposited in or computed by PubChem | |
DSSTOX Substance ID |
DTXSID001043100 | |
Record name | Benzmalecene | |
Source | EPA DSSTox | |
URL | https://comptox.epa.gov/dashboard/DTXSID001043100 | |
Description | DSSTox provides a high quality public chemistry resource for supporting improved predictive toxicology. | |
Molecular Weight |
392.3 g/mol | |
Source | PubChem | |
URL | https://pubchem.ncbi.nlm.nih.gov | |
Description | Data deposited in or computed by PubChem | |
CAS No. |
148-07-2 | |
Record name | Benzmalecene [INN] | |
Source | ChemIDplus | |
URL | https://pubchem.ncbi.nlm.nih.gov/substance/?source=chemidplus&sourceid=0000148072 | |
Description | ChemIDplus is a free, web search system that provides access to the structure and nomenclature authority files used for the identification of chemical substances cited in National Library of Medicine (NLM) databases, including the TOXNET system. | |
Record name | Benzmalecene | |
Source | EPA DSSTox | |
URL | https://comptox.epa.gov/dashboard/DTXSID001043100 | |
Description | DSSTox provides a high quality public chemistry resource for supporting improved predictive toxicology. | |
Record name | BENZMALECENE | |
Source | FDA Global Substance Registration System (GSRS) | |
URL | https://gsrs.ncats.nih.gov/ginas/app/beta/substances/6ET4K804XA | |
Description | The FDA Global Substance Registration System (GSRS) enables the efficient and accurate exchange of information on what substances are in regulated products. Instead of relying on names, which vary across regulatory domains, countries, and regions, the GSRS knowledge base makes it possible for substances to be defined by standardized, scientific descriptions. | |
Explanation | Unless otherwise noted, the contents of the FDA website (www.fda.gov), both text and graphics, are not copyrighted. They are in the public domain and may be republished, reprinted and otherwise used freely by anyone without the need to obtain permission from FDA. Credit to the U.S. Food and Drug Administration as the source is appreciated but not required. | |
Retrosynthesis Analysis
AI-Powered Synthesis Planning: Our tool employs the Template_relevance Pistachio, Template_relevance Bkms_metabolic, Template_relevance Pistachio_ringbreaker, Template_relevance Reaxys, Template_relevance Reaxys_biocatalysis model, leveraging a vast database of chemical reactions to predict feasible synthetic routes.
One-Step Synthesis Focus: Specifically designed for one-step synthesis, it provides concise and direct routes for your target compounds, streamlining the synthesis process.
Accurate Predictions: Utilizing the extensive PISTACHIO, BKMS_METABOLIC, PISTACHIO_RINGBREAKER, REAXYS, REAXYS_BIOCATALYSIS database, our tool offers high-accuracy predictions, reflecting the latest in chemical research and data.
Strategy Settings
Precursor scoring | Relevance Heuristic |
---|---|
Min. plausibility | 0.01 |
Model | Template_relevance |
Template Set | Pistachio/Bkms_metabolic/Pistachio_ringbreaker/Reaxys/Reaxys_biocatalysis |
Top-N result to add to graph | 6 |
Feasible Synthetic Routes
試験管内研究製品の免責事項と情報
BenchChemで提示されるすべての記事および製品情報は、情報提供を目的としています。BenchChemで購入可能な製品は、生体外研究のために特別に設計されています。生体外研究は、ラテン語の "in glass" に由来し、生物体の外で行われる実験を指します。これらの製品は医薬品または薬として分類されておらず、FDAから任何の医療状態、病気、または疾患の予防、治療、または治癒のために承認されていません。これらの製品を人間または動物に体内に導入する形態は、法律により厳格に禁止されています。これらのガイドラインに従うことは、研究と実験において法的および倫理的な基準の遵守を確実にするために重要です。