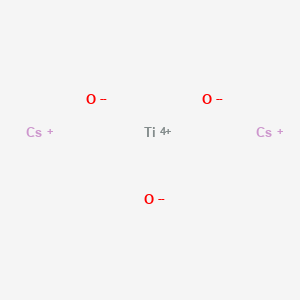
チタン酸セシウム
説明
科学的研究の応用
Cesium titanate has a wide range of scientific research applications:
Medicine: Research is ongoing into its potential use in medical applications, particularly in the development of materials for radiation therapy.
作用機序
Cesium titanate, also known as dicesium;oxygen(2-);titanium(4+), is an inorganic compound with the formula Cs2TiO3 . It has been studied for its potential applications in various fields, particularly in environmental remediation and energy storage .
Target of Action
The primary target of cesium titanate is cesium ions in aqueous solutions . Cesium ions are a significant concern in the environment due to their radioactive isotopes, which can cause harmful radiation to organisms .
Mode of Action
Cesium titanate interacts with its targets through ion exchange . Specifically, the compound has a high efficiency in ion exchange, allowing it to adsorb cesium ions from aqueous solutions . This interaction results in the removal of cesium ions from the solution, thereby reducing their potential harm .
Biochemical Pathways
By reducing the concentration of radioactive cesium in the environment, it can help prevent the cellular damage that these ions can cause, which may lead to diseases such as cancer and genetic mutations .
Pharmacokinetics
It’s worth noting that cesium titanate is insoluble in water , which could impact its distribution and elimination in an environmental context.
Result of Action
The primary result of cesium titanate’s action is the removal of cesium ions from aqueous solutions . Studies have shown that it can remove about 88% of Cs(I) when certain conditions are met . This high removal rate demonstrates the compound’s effectiveness in adsorbing cesium ions .
Action Environment
The action of cesium titanate is influenced by various environmental factors. For instance, the pH of the solution plays a significant role in the adsorption process of Cs(I) . Moreover, the compound exhibits excellent selectivity for Cs(I) in the presence of common cations, suggesting that it can effectively function even in complex environmental mixtures .
生化学分析
Biochemical Properties
Cesium titanate is known for its high efficiency in ion exchange . This property allows it to interact with various biomolecules, particularly metal ions, in biochemical reactions . The main interaction mechanism of cesium titanate with these ions is ion exchange .
Cellular Effects
The presence of cesium in the environment can cause harmful radiation to organisms, leading to permanent damage at the cellular level . This damage may result in diseases such as cancer, genetic mutations, and genetic diseases . Cesium titanate, due to its ion exchange properties, can effectively adsorb Cs-containing wastewater, thereby reducing the potential harm caused by cesium .
Molecular Mechanism
The molecular mechanism of action of cesium titanate primarily involves ion exchange . This process involves the exchange of cesium ions with other ions, particularly metal ions . This ion exchange mechanism is crucial for the compound’s ability to adsorb Cs-containing wastewater .
Temporal Effects in Laboratory Settings
In laboratory settings, the adsorption equilibrium of cesium ions by cesium titanate is reached in about 20 minutes . The compound exhibits a high adsorption capacity for Cs (I), with a maximum adsorption capacity of 200.00 mg/g .
Metabolic Pathways
Its primary known function is in ion exchange, particularly with metal ions
Transport and Distribution
Its ion exchange properties suggest that it may interact with various transporters or binding proteins .
準備方法
Synthetic Routes and Reaction Conditions: Cesium titanate can be synthesized through solid-state reactions. One common method involves heating a mixture of cesium carbonate (Cs₂CO₃) and anatase titanium dioxide (TiO₂) at a molar ratio of 1:5 at 1000°C for 20 hours . This process yields Cs₂Ti₅O₁₁, a layered cesium titanate.
Industrial Production Methods: Industrial production of cesium titanate typically follows similar solid-state reaction methods, with precise control over temperature and reaction time to ensure the desired phase and purity of the product. Mechanochemical reactions, involving the grinding of reactants in an agate mortar and pestle, are also employed to produce various cesium titanate compounds .
化学反応の分析
Types of Reactions: Cesium titanate primarily undergoes ion exchange reactions. It can exchange its cesium ions with other cations such as hydrogen, lithium, and sodium in aqueous solutions . This ion exchange capability is crucial for its applications in removing cesium from contaminated water.
Common Reagents and Conditions: The ion exchange reactions typically occur in aqueous solutions, with the pH and concentration of the solution playing significant roles. For example, the removal rate of cesium ions is about 88% when the pH is 5.00 ± 0.05, with a cesium concentration of 10 ppm and a titanate nanosheet concentration of 0.1 g/L .
Major Products Formed: The major products formed from these ion exchange reactions are the exchanged titanate compounds, such as hydrogen titanate, lithium titanate, and sodium titanate .
類似化合物との比較
Barium titanate (BaTiO₃): Like cesium titanate, barium titanate is an inorganic compound with a polymeric structure. It is widely used in capacitors, piezoelectric devices, and as a dielectric material.
Iron(II) titanate (FeTiO₃): This compound is another titanate with similar structural properties but different applications, primarily in pigments and as a precursor for other iron compounds.
Uniqueness of Cesium Titanate: Cesium titanate’s unique ability to selectively exchange cesium ions makes it particularly valuable for applications in radioactive waste treatment. Its high ion exchange capacity and selectivity for cesium ions set it apart from other titanates, making it a preferred choice for cesium ion removal in various environmental and industrial contexts .
生物活性
Cesium titanate (CsTiO₃) is a compound that has garnered attention for its potential applications in various fields, including environmental remediation, photocatalysis, and biomedical applications. This article provides a detailed examination of the biological activity associated with cesium titanate, highlighting its interactions with biological systems, cytotoxicity, and potential therapeutic uses.
Cesium titanate can be synthesized through various methods, including solid-state reactions and hydrothermal processes. The chemical formula CsTiO₃ indicates that it consists of cesium ions and titanium oxide, forming a perovskite structure that is stable under various conditions. Its unique properties stem from this structure, which allows for ion exchange and photocatalytic activity.
Cytotoxicity Studies
Research has indicated that cesium titanate exhibits varying degrees of cytotoxicity depending on the concentration and exposure duration. For instance, a study showed that cesium titanate nanoparticles had minimal cytotoxic effects on human cell lines at lower concentrations but demonstrated significant cytotoxicity at higher doses. This suggests a dose-dependent relationship where lower concentrations may be safe for cellular interactions while higher concentrations could induce cell death.
Table 1: Cytotoxicity of Cesium Titanate on Different Cell Lines
Cell Line | Concentration (µg/mL) | Cell Viability (%) | Observations |
---|---|---|---|
HeLa | 10 | 95 | Minimal effect |
HeLa | 100 | 70 | Moderate cytotoxicity |
L929 | 10 | 90 | Minimal effect |
L929 | 100 | 50 | Significant cytotoxicity |
Inflammatory Response
The interaction of cesium titanate with immune cells has been studied to understand its potential as a therapeutic agent. In vitro experiments revealed that cesium titanate could modulate inflammatory responses in monocytes. When exposed to bacterial lipopolysaccharides (LPS), cells treated with cesium titanate exhibited altered cytokine secretion profiles, indicating a potential role in immune modulation.
Case Study: Modulation of Cytokine Secretion
In a study involving THP-1 monocytes, cesium titanate was shown to enhance the secretion of TNF-α when activated by LPS. This suggests that cesium titanate may have immunomodulatory properties that could be harnessed in therapeutic applications.
Photocatalytic Activity and Environmental Applications
Cesium titanate is also noted for its photocatalytic properties, particularly in the degradation of organic pollutants. Studies have demonstrated that cesium titanate can effectively degrade dyes and other organic compounds under UV light irradiation. This property is attributed to its ability to generate reactive oxygen species (ROS), which facilitate the breakdown of complex organic molecules.
Table 2: Photocatalytic Efficiency of Cesium Titanate
Pollutant | Initial Concentration (mg/L) | Degradation Rate (%) | Reaction Time (min) |
---|---|---|---|
Methylene Blue | 10 | 85 | 60 |
Rhodamine B | 10 | 90 | 60 |
Phenol | 5 | 75 | 30 |
Ion Exchange Properties
The ion-exchange capacity of cesium titanate makes it an effective material for removing cesium ions from contaminated water sources. Research indicates that cesium titanate can achieve high removal rates through ion exchange mechanisms, making it a viable candidate for environmental remediation efforts.
Case Study: Removal of Cesium Ions from Water
A study demonstrated that cesium titanate nanosheets could remove up to 88% of Cs⁺ ions from aqueous solutions within 20 minutes, showcasing its potential as an adsorbent for radioactive waste management.
特性
IUPAC Name |
dicesium;oxygen(2-);titanium(4+) | |
---|---|---|
Source | PubChem | |
URL | https://pubchem.ncbi.nlm.nih.gov | |
Description | Data deposited in or computed by PubChem | |
InChI |
InChI=1S/2Cs.3O.Ti/q2*+1;3*-2;+4 | |
Source | PubChem | |
URL | https://pubchem.ncbi.nlm.nih.gov | |
Description | Data deposited in or computed by PubChem | |
InChI Key |
UKGZHELIUYCPTO-UHFFFAOYSA-N | |
Source | PubChem | |
URL | https://pubchem.ncbi.nlm.nih.gov | |
Description | Data deposited in or computed by PubChem | |
Canonical SMILES |
[O-2].[O-2].[O-2].[Ti+4].[Cs+].[Cs+] | |
Source | PubChem | |
URL | https://pubchem.ncbi.nlm.nih.gov | |
Description | Data deposited in or computed by PubChem | |
Molecular Formula |
Cs2O3Ti | |
Source | PubChem | |
URL | https://pubchem.ncbi.nlm.nih.gov | |
Description | Data deposited in or computed by PubChem | |
DSSTOX Substance ID |
DTXSID10923872 | |
Record name | Cesium titanium oxide (Cs2TiO3) | |
Source | EPA DSSTox | |
URL | https://comptox.epa.gov/dashboard/DTXSID10923872 | |
Description | DSSTox provides a high quality public chemistry resource for supporting improved predictive toxicology. | |
Molecular Weight |
361.676 g/mol | |
Source | PubChem | |
URL | https://pubchem.ncbi.nlm.nih.gov | |
Description | Data deposited in or computed by PubChem | |
CAS No. |
12158-57-5 | |
Record name | Cesium titanium oxide (Cs2TiO3) | |
Source | ChemIDplus | |
URL | https://pubchem.ncbi.nlm.nih.gov/substance/?source=chemidplus&sourceid=0012158575 | |
Description | ChemIDplus is a free, web search system that provides access to the structure and nomenclature authority files used for the identification of chemical substances cited in National Library of Medicine (NLM) databases, including the TOXNET system. | |
Record name | Cesium titanium oxide (Cs2TiO3) | |
Source | EPA DSSTox | |
URL | https://comptox.epa.gov/dashboard/DTXSID10923872 | |
Description | DSSTox provides a high quality public chemistry resource for supporting improved predictive toxicology. | |
試験管内研究製品の免責事項と情報
BenchChemで提示されるすべての記事および製品情報は、情報提供を目的としています。BenchChemで購入可能な製品は、生体外研究のために特別に設計されています。生体外研究は、ラテン語の "in glass" に由来し、生物体の外で行われる実験を指します。これらの製品は医薬品または薬として分類されておらず、FDAから任何の医療状態、病気、または疾患の予防、治療、または治癒のために承認されていません。これらの製品を人間または動物に体内に導入する形態は、法律により厳格に禁止されています。これらのガイドラインに従うことは、研究と実験において法的および倫理的な基準の遵守を確実にするために重要です。