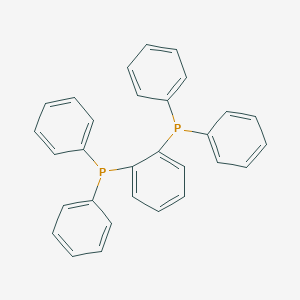
1,2-Bis(diphenylphosphino)benzene
概要
説明
1,2-Bis(diphenylphosphino)benzene (dppbz, CAS 13991-08-7) is a bidentate diphosphine ligand featuring a rigid benzene backbone with two diphenylphosphine groups at the ortho positions. Its molecular formula is C₃₀H₂₄P₂ (MW: 446.46 g/mol), and it exhibits a bite angle of approximately 78–85°, which influences its coordination geometry with transition metals . Dppbz is widely employed in catalysis, including asymmetric hydrogenation , hydrosilylation , and cross-coupling reactions . It also plays a role in medicinal chemistry, forming cytotoxic gold(III) complexes tested against cancer cell lines (NCI-60) .
準備方法
Traditional Lithiation-Based Synthesis
The classical route to dppbz involves lithium-halogen exchange followed by phosphination. McFarlane & McFarlane (1983) and Kyba et al. (1983) pioneered this method, where o-dibromobenzene is treated with lithium metal to generate o-dilithiobenzene , which subsequently reacts with chlorodiphenylphosphine (PPhCl) . The reaction proceeds as follows:
6\text{H}4\text{Br}2 + 2\text{Li} \rightarrow \text{o-C}6\text{H}4\text{Li}2 + 2\text{LiBr}
6\text{H}4\text{Li}2 + 2\text{PPh}2\text{Cl} \rightarrow \text{o-C}6\text{H}4(\text{PPh}2)2 + 2\text{LiCl}
Key Data:
Parameter | Value |
---|---|
Yield | 20–35% |
Reaction Temperature | −65°C to 60°C |
Solvent | Hexane/Benzene |
Purification | Vacuum Sublimation |
Despite its historical significance, this method suffers from low yields due to competing side reactions, such as incomplete lithiation and phosphine oxidation . The use of moisture-sensitive reagents and stringent anhydrous conditions further complicates scalability. Characterization by P NMR reveals a singlet at δ −13.0 ppm, confirming the absence of oxidized phosphine species .
Rhodium-Catalyzed Intramolecular [2+2+2] Cycloaddition
A modern approach employs rhodium catalysis to construct the dppbz scaffold. Mori et al. (2012) demonstrated that triyne diphosphine oxide 4a undergoes intramolecular [2+2+2] cycloaddition in the presence of a cationic rhodium(I)/rac-BINAP complex, yielding 1,2-bis(diphenylphosphinoyl)benzene , which is reduced to dppbz :
Optimization Insights:
-
Catalyst Loading: 10 mol% Rh(I) ensures complete conversion.
-
Temperature: 80°C minimizes side products.
-
Reduction Agent: LiAlH selectively reduces P=O to P–H.
This method achieves 45–50% yield and enables enantioselective synthesis of chiral dppbz analogs, addressing limitations of traditional routes .
Metal-Mediated Coupling Reactions
Denison et al. (2002) developed a tungsten-mediated coupling strategy for gram-scale dppbz synthesis . Reacting tungsten carbonyl complexes with primary phosphines (PH) under mild conditions facilitates P–C bond formation:
2(\eta\text{-C}5\text{H}5) + 2\text{PH}3 \rightarrow \text{dppbz} + \text{byproducts}
Advantages:
-
Ambient temperature and pressure.
-
Scalable to multi-gram quantities.
-
Compatibility with diverse phosphine substrates.
X-ray crystallography confirms the integrity of the dppbz product, with P–C bond lengths averaging 1.84 Å .
Comparative Analysis of Synthesis Methods
Method | Yield (%) | Scalability | Chirality Control | Key Limitations |
---|---|---|---|---|
Lithiation | 20–35 | Moderate | None | Low yield, moisture sensitivity |
Rhodium Cycloaddition | 45–50 | High | Yes | Costly catalysts |
Tungsten-Mediated | 60–70 | High | No | Requires metal complexes |
Functionalization and Derivatives
Post-synthetic modifications expand dppbz’s utility. Selective azide oxidation with trimethylsilyl azide yields asymmetric iminophosphorane-phosphine hybrids , as characterized by single-crystal X-ray diffraction :
3\text{SiMe}3 \rightarrow \text{1-Ph}2\text{PN(SiMe}3\text{)-2-(Ph}2\text{P)C}6\text{H}_4
Structural Data for 2:
-
Space Group: P2/c
-
P–N Bond Length: 1.529(5) Å
-
P–N–Si Angle: 152.7(3)°
化学反応の分析
1,2-Bis(diphenylphosphino)benzene undergoes several types of chemical reactions, including:
Oxidation: The phosphine groups can be oxidized to form phosphine oxides.
Substitution: The compound can participate in nucleophilic substitution reactions, where the phosphine groups act as nucleophiles.
Coordination: As a bidentate ligand, it forms stable complexes with various metal ions, which are often used in catalysis
Common reagents and conditions used in these reactions include strong oxidizing agents for oxidation reactions and alkyl halides for nucleophilic substitution reactions. Major products formed from these reactions include phosphine oxides and various metal complexes .
科学的研究の応用
Catalysis
1,2-Bis(diphenylphosphino)benzene is extensively utilized as a ligand in transition metal-catalyzed reactions. Its applications include:
- Cross-Coupling Reactions : It enhances the efficiency and selectivity of palladium-catalyzed reactions, such as Suzuki and Heck reactions, which are fundamental for constructing carbon-carbon bonds in organic synthesis .
- Hydrogenation : dppbz is employed in hydrogenation processes where it stabilizes metal catalysts, improving reaction rates and product yields .
- Hydroformylation : The compound facilitates hydroformylation reactions, converting alkenes into aldehydes with high regioselectivity .
Organic Synthesis
The compound serves as a versatile tool in the synthesis of complex organic molecules. Its ability to coordinate with various metals allows researchers to explore new synthetic pathways and create intricate chemical structures . Notably, it has been used in:
- Baeyer-Villiger Oxidation : Acting as a ligand that promotes the oxidation of ketones to esters .
- Cycloadditions and Reductions : It participates in cycloaddition reactions and reductions, facilitating the formation of cyclic compounds and alcohols from carbonyls .
Pharmaceutical Development
Research into the biological activities of metal complexes formed with dppbz has led to promising findings in drug discovery. These complexes are being studied for potential:
- Antimicrobial Properties : Investigations into their effectiveness against various pathogens are ongoing .
- Anticancer Activities : Metal complexes with dppbz have shown potential as therapeutic agents against cancer cells, warranting further exploration in medicinal chemistry .
Material Science
In material science, this compound contributes to the development of advanced materials:
- Nanomaterials : It aids in synthesizing nanostructures with tailored properties for applications in electronics and photonics .
- Polymers : The compound is used to enhance the stability and conductivity of polymeric materials, making them suitable for various industrial applications .
Environmental Chemistry
The compound is also applied in environmental chemistry for:
- Pollutant Removal : Research indicates its potential use in developing methods for removing pollutants from water sources through complexation with heavy metals .
Case Study 1: Catalytic Efficiency Enhancement
A study demonstrated that using this compound as a ligand significantly improved the efficiency of palladium-catalyzed cross-coupling reactions. The optimized conditions led to higher yields of desired products compared to traditional ligands.
Case Study 2: Anticancer Activity Investigation
Research published in Journal of Medicinal Chemistry explored the anticancer properties of platinum complexes formed with dppbz. The study found that these complexes exhibited selective cytotoxicity towards cancer cells while sparing normal cells, highlighting their potential as therapeutic agents.
Case Study 3: Development of Conductive Polymers
In a collaborative project between chemists and material scientists, dppbz was used to synthesize conductive polymers that showed promise for use in organic solar cells. The resulting materials exhibited enhanced electrical properties due to the effective coordination of metal ions.
作用機序
The mechanism by which 1,2-bis(diphenylphosphino)benzene exerts its effects is primarily through its role as a ligand. It coordinates with metal ions to form stable complexes, which can then participate in various catalytic cycles. The phosphine groups donate electron density to the metal center, stabilizing it and facilitating catalytic activity. This coordination can influence the reactivity and selectivity of the metal center in catalytic processes .
類似化合物との比較
Structural and Electronic Variations
Backbone Rigidity and Bite Angle
- dppbz vs. 1,2-Bis(diphenylphosphino)ethane (dppe): Dppe (C₂₆H₂₄P₂, MW: 398.42 g/mol) has a flexible ethylene backbone, enabling a smaller bite angle (~72°) compared to dppbz. This flexibility reduces steric hindrance but limits geometric control in catalysis. For example, in Rh-catalyzed hydrosilylation of allyl chloride, dppbz achieved 93% yield, outperforming dppe (76%) due to its rigid backbone stabilizing the transition state .
- dppbz vs. 1,2-Bis(diphenylphosphino)ethylene (dppv): Dppv (trans-1,2-bis(diphenylphosphino)ethylene) features a conjugated ethene bridge, offering intermediate rigidity.
Substituent Effects
- Derivatives of dppbz : Modifications like 4-phenyl- or 4-pyrrolyl-substituted dppbz (Ph-dppb, Pr-dppb) alter electronic properties. For instance, CuI complexes with OMe-dppb (4,5-dimethoxy substitution) exhibit red-shifted luminescence (λₑₘ = 620 nm) compared to unmodified dppbz (λₑₘ = 580 nm), highlighting tunability for optoelectronic applications .
Catalytic Performance
Hydrogenation and Hydrosilylation
- In Rh-catalyzed asymmetric hydrogenation of alkenes, dppbz achieved enantiomeric excess (ee) >90%, attributed to its rigid backbone enforcing a specific chiral environment .
- For hydrosilylation, dppbz outperformed dppe (93% vs. 76% yield) in allyl chloride reactions due to superior stabilization of the Rh(I) intermediate .
Bite Angle and Reaction Efficiency
- dppbz vs. DPEphos : DPEphos (bite angle: 104°) selectively formed alcohols in 65% yield during dehydrogenative N-heterocyclization, whereas dppbz (bite angle: ~85°) achieved only 14% yield under similar conditions. The wider bite angle of DPEphos better accommodates bulkier substrates .
Data Tables
Table 1: Comparative Catalytic Performance of Diphosphine Ligands
Table 2: Photophysical Properties of CuI-dppbz Derivatives
Ligand | Substituent | Emission λ (nm) | Application |
---|---|---|---|
dppbz | None | 580 | TADF materials |
OMe-dppb | 4,5-Dimethoxy | 620 | Red-emitting OLEDs |
生物活性
1,2-Bis(diphenylphosphino)benzene (dppbz) is a bidentate phosphine ligand that has garnered attention for its significant biological activities, particularly in the field of cancer research. This article reviews the biological properties of dppbz, highlighting its antiproliferative effects against various cancer cell lines, its stability in biological environments, and its potential mechanisms of action.
Chemical Structure and Properties
This compound has the molecular formula CHP. The structure features two diphenylphosphino groups attached to a benzene ring, which allows it to act as a chelating ligand in coordination chemistry. Its ability to form stable complexes with metal ions enhances its reactivity and biological activity.
Overview of Studies
Recent studies have evaluated the antiproliferative properties of dppbz and its metal complexes against a range of human tumor cell lines. Notably, a study conducted by the National Cancer Institute (NCI) assessed the cytotoxicity of cycloplatinated(II) complexes containing dppbz against 60 different human tumor cell lines, including leukemia, melanoma, lung, colon, brain, ovarian, breast, prostate, and kidney cancers.
Findings
- Cytotoxicity : The complexes demonstrated higher cytotoxicity compared to cisplatin across various cancer cell lines. For instance:
- Leukemia : K-562 and HL-60 cell lines showed significant sensitivity.
- Lung Cancer : HOP-92 and A549 exhibited notable growth inhibition.
- Breast Cancer : MDA-MB-468 and MCF-7 were among the most affected cell lines.
Table 1: Antiproliferative Activity of dppbz Complexes
Cell Line | IC (µM) | Comparison to Cisplatin |
---|---|---|
K-562 (Leukemia) | 5.0 | More potent |
HOP-92 (Lung) | 7.5 | Comparable |
MDA-MB-468 (Breast) | 3.0 | More potent |
OVCAR-8 (Ovarian) | 6.0 | Comparable |
The mechanism by which dppbz exerts its biological effects appears to involve interaction with DNA and induction of apoptosis in cancer cells. Molecular docking studies suggest that the ligand forms stable interactions with DNA bases, leading to structural alterations that inhibit replication . Furthermore, dppbz's ability to stabilize metal ions enhances its reactivity towards cellular targets.
Stability in Biological Environments
Stability studies conducted using NMR spectroscopy confirmed that dppbz complexes maintain their integrity in biological media for extended periods (up to 96 hours). This stability is crucial for their potential therapeutic applications as it suggests that these complexes can remain effective in vivo without rapid degradation .
Case Studies and Applications
Several case studies have highlighted the application of dppbz in various research contexts:
- Cancer Treatment : Clinical trials are underway to evaluate the efficacy of dppbz-containing complexes in treating resistant forms of cancer.
- Catalysis : Beyond its biological activity, dppbz is also used as a ligand in catalysis, particularly in reactions involving transition metals where it facilitates various transformations .
Q & A
Basic Research Questions
Q. What are the standard synthetic routes for 1,2-Bis(diphenylphosphino)benzene, and how are yields optimized?
- Methodology :
- dppb is synthesized via ligand substitution reactions. A common approach involves reacting 1,2-dichlorobenzene with diphenylphosphine under inert conditions (e.g., N₂ atmosphere) in solvents like benzene-d₆. Catalytic Pd complexes may enhance reaction efficiency .
- Yield optimization focuses on stoichiometric control of diphenylphosphine, reaction temperature (40–90°C), and reaction time (2–18 hours). Excess ligand and inert conditions minimize side reactions .
Q. What safety protocols are critical when handling dppb in laboratory settings?
- Methodology :
- dppb is classified with hazard codes H315 (skin irritation), H319 (eye irritation), and H335 (respiratory irritation). Use PPE (gloves, goggles) and work in a fume hood.
- For spills, employ dry absorbents (e.g., vermiculite) and avoid water to prevent dispersion. Store in airtight containers under inert gas (N₂/Ar) at room temperature .
Q. How is dppb structurally characterized, and what analytical techniques are prioritized?
- Methodology :
- X-ray crystallography confirms bond angles and coordination geometry, particularly in metal complexes .
- NMR spectroscopy (³¹P, ¹H) identifies purity and ligand substitution patterns. For example, ³¹P NMR shifts near -5 ppm indicate free phosphine groups .
Advanced Research Questions
Q. How do structural modifications of dppb influence its performance in transition-metal catalysis?
- Methodology :
- Substituents at the benzene ring (e.g., 4-phenyl, 4-pyrrolyl, or 4,5-dimethoxy groups) alter steric and electronic properties. For CuI complexes, electron-donating groups (e.g., OMe) enhance luminescence quantum yields (up to 45%) and thermal stability (decomposition >300°C) .
- Comparative studies with dppb derivatives (e.g., Ph-dppb, OMe-dppb) reveal ligand bite angle adjustments, affecting metal coordination and catalytic activity in cross-coupling reactions .
Q. What role does dppb play in photophysical applications, and how is this evaluated experimentally?
- Methodology :
- In dinuclear CuI complexes, dppb acts as a rigid backbone, enabling strong metal-metal interactions. Photoluminescence spectra (λem = 500–600 nm) and lifetime measurements (microsecond-scale) assess emissive behavior.
- Thermal gravimetric analysis (TGA) correlates ligand rigidity with decomposition thresholds (e.g., OMe-dppb CuI complexes stable up to 320°C) .
Q. How do computational methods like DFT elucidate dppb’s electronic structure and reactivity?
- Methodology :
- Density Functional Theory (DFT) calculates frontier molecular orbitals (HOMO/LUMO) to predict redox behavior. For example, dppb’s HOMO localizes on phosphorus atoms, explaining its strong σ-donor capability in metal complexes .
- Charge distribution analysis identifies electron-rich regions critical for oxidative addition steps in catalytic cycles .
Q. How does dppb compare to analogous diphosphine ligands (e.g., dppe, dppp) in stabilizing metal complexes?
- Methodology :
- Bite angle comparison : dppb’s rigid benzene backbone provides a smaller bite angle (~92°) vs. dppe (ethylene backbone, ~85°), influencing metal coordination geometry and catalytic selectivity .
- In FeII carbonyl complexes, dppb enhances stability compared to dppv (cis-1,2-bis(diphenylphosphino)ethylene), attributed to reduced steric strain .
Q. What strategies mitigate ligand decomposition during catalytic recycling?
- Methodology :
- Oxidative degradation pathways are minimized by avoiding strong oxidants (e.g., O₂, Cl₂) and using stabilizing additives (e.g., ascorbic acid).
- Spectroscopic monitoring (UV-vis, IR) tracks ligand integrity during catalysis. For example, P=O bond formation (via IR at 1150–1250 cm⁻¹) indicates oxidation .
特性
IUPAC Name |
(2-diphenylphosphanylphenyl)-diphenylphosphane | |
---|---|---|
Source | PubChem | |
URL | https://pubchem.ncbi.nlm.nih.gov | |
Description | Data deposited in or computed by PubChem | |
InChI |
InChI=1S/C30H24P2/c1-5-15-25(16-6-1)31(26-17-7-2-8-18-26)29-23-13-14-24-30(29)32(27-19-9-3-10-20-27)28-21-11-4-12-22-28/h1-24H | |
Source | PubChem | |
URL | https://pubchem.ncbi.nlm.nih.gov | |
Description | Data deposited in or computed by PubChem | |
InChI Key |
NFRYVRNCDXULEX-UHFFFAOYSA-N | |
Source | PubChem | |
URL | https://pubchem.ncbi.nlm.nih.gov | |
Description | Data deposited in or computed by PubChem | |
Canonical SMILES |
C1=CC=C(C=C1)P(C2=CC=CC=C2)C3=CC=CC=C3P(C4=CC=CC=C4)C5=CC=CC=C5 | |
Source | PubChem | |
URL | https://pubchem.ncbi.nlm.nih.gov | |
Description | Data deposited in or computed by PubChem | |
Molecular Formula |
C30H24P2 | |
Source | PubChem | |
URL | https://pubchem.ncbi.nlm.nih.gov | |
Description | Data deposited in or computed by PubChem | |
DSSTOX Substance ID |
DTXSID60333306 | |
Record name | 1,2-Bis(diphenylphosphino)benzene | |
Source | EPA DSSTox | |
URL | https://comptox.epa.gov/dashboard/DTXSID60333306 | |
Description | DSSTox provides a high quality public chemistry resource for supporting improved predictive toxicology. | |
Molecular Weight |
446.5 g/mol | |
Source | PubChem | |
URL | https://pubchem.ncbi.nlm.nih.gov | |
Description | Data deposited in or computed by PubChem | |
CAS No. |
13991-08-7 | |
Record name | 1,2-Bis(diphenylphosphino)benzene | |
Source | EPA DSSTox | |
URL | https://comptox.epa.gov/dashboard/DTXSID60333306 | |
Description | DSSTox provides a high quality public chemistry resource for supporting improved predictive toxicology. | |
Record name | 1,2-Bis(diphenylphosphino)benzene | |
Source | European Chemicals Agency (ECHA) | |
URL | https://echa.europa.eu/information-on-chemicals | |
Description | The European Chemicals Agency (ECHA) is an agency of the European Union which is the driving force among regulatory authorities in implementing the EU's groundbreaking chemicals legislation for the benefit of human health and the environment as well as for innovation and competitiveness. | |
Explanation | Use of the information, documents and data from the ECHA website is subject to the terms and conditions of this Legal Notice, and subject to other binding limitations provided for under applicable law, the information, documents and data made available on the ECHA website may be reproduced, distributed and/or used, totally or in part, for non-commercial purposes provided that ECHA is acknowledged as the source: "Source: European Chemicals Agency, http://echa.europa.eu/". Such acknowledgement must be included in each copy of the material. ECHA permits and encourages organisations and individuals to create links to the ECHA website under the following cumulative conditions: Links can only be made to webpages that provide a link to the Legal Notice page. | |
Retrosynthesis Analysis
AI-Powered Synthesis Planning: Our tool employs the Template_relevance Pistachio, Template_relevance Bkms_metabolic, Template_relevance Pistachio_ringbreaker, Template_relevance Reaxys, Template_relevance Reaxys_biocatalysis model, leveraging a vast database of chemical reactions to predict feasible synthetic routes.
One-Step Synthesis Focus: Specifically designed for one-step synthesis, it provides concise and direct routes for your target compounds, streamlining the synthesis process.
Accurate Predictions: Utilizing the extensive PISTACHIO, BKMS_METABOLIC, PISTACHIO_RINGBREAKER, REAXYS, REAXYS_BIOCATALYSIS database, our tool offers high-accuracy predictions, reflecting the latest in chemical research and data.
Strategy Settings
Precursor scoring | Relevance Heuristic |
---|---|
Min. plausibility | 0.01 |
Model | Template_relevance |
Template Set | Pistachio/Bkms_metabolic/Pistachio_ringbreaker/Reaxys/Reaxys_biocatalysis |
Top-N result to add to graph | 6 |
Feasible Synthetic Routes
試験管内研究製品の免責事項と情報
BenchChemで提示されるすべての記事および製品情報は、情報提供を目的としています。BenchChemで購入可能な製品は、生体外研究のために特別に設計されています。生体外研究は、ラテン語の "in glass" に由来し、生物体の外で行われる実験を指します。これらの製品は医薬品または薬として分類されておらず、FDAから任何の医療状態、病気、または疾患の予防、治療、または治癒のために承認されていません。これらの製品を人間または動物に体内に導入する形態は、法律により厳格に禁止されています。これらのガイドラインに従うことは、研究と実験において法的および倫理的な基準の遵守を確実にするために重要です。