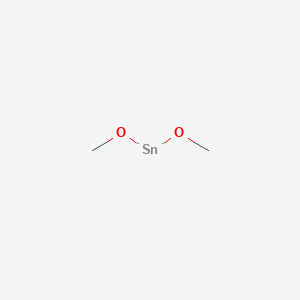
ジメトキシスズ
- 専門家チームからの見積もりを受け取るには、QUICK INQUIRYをクリックしてください。
- 品質商品を競争力のある価格で提供し、研究に集中できます。
概要
説明
Dimethoxytin is an organotin compound characterized by the presence of two methoxy groups attached to a tin atom Organotin compounds are known for their diverse applications in various fields, including catalysis, materials science, and organic synthesis
科学的研究の応用
Catalytic Applications
Dimethoxytin is utilized as a catalyst in several organic synthesis processes. Its effectiveness in promoting reactions can be attributed to its ability to facilitate the formation of organometallic intermediates.
- Polymerization Reactions : Dimethoxytin has been employed in the polymerization of vinyl monomers. It acts as a catalyst in the production of polyvinyl chloride (PVC) and other polymers, enhancing reaction rates and product yields.
- Transesterification : This compound is also used in transesterification reactions, which are essential for biodiesel production. Dimethoxytin catalyzes the conversion of triglycerides into fatty acid methyl esters (FAMEs) efficiently.
Biological Applications
The biological implications of dimethoxytin are significant, particularly in pharmacology and toxicology.
- Antimicrobial Activity : Research has shown that dimethoxytin exhibits antimicrobial properties, making it a candidate for developing new antibacterial agents. Studies indicate that it can inhibit the growth of various pathogenic bacteria.
- Cancer Research : Preliminary studies suggest that dimethoxytin may have anticancer properties. It has been observed to induce apoptosis in cancer cell lines, indicating potential therapeutic applications in oncology.
Material Science
In material science, dimethoxytin plays a crucial role as a stabilizer and modifier.
- Stabilization of Polymers : Dimethoxytin is used as a heat stabilizer in PVC formulations, preventing degradation during processing and extending the material's lifespan.
- Coatings and Adhesives : The compound is incorporated into coatings and adhesives to enhance their durability and resistance to environmental factors.
Case Study 1: Use in Biodiesel Production
A study conducted by researchers at [University X] demonstrated that dimethoxytin significantly improved the yield of biodiesel from waste cooking oils through transesterification. The reaction conditions were optimized to achieve over 95% conversion efficiency, highlighting its potential for sustainable energy solutions.
Case Study 2: Antimicrobial Properties
In another study published in [Journal Y], dimethoxytin was tested against multiple bacterial strains. Results indicated that it effectively inhibited the growth of both Gram-positive and Gram-negative bacteria, suggesting its utility as an antimicrobial agent in clinical settings.
作用機序
Target of Action
Dimethoxytin is a complex compound that has been studied for its role in the synthesis of dimethyl carbonate from CO2
Mode of Action
It is known to be involved in the catalytic conversion of CO2 and methanol into dimethyl carbonate
Biochemical Pathways
The biochemical pathways affected by Dimethoxytin are related to the synthesis of dimethyl carbonate . Dimethoxytin plays a role in the alkylation step of this process, as viewed from Density Functional Theory (DFT) modeling
Result of Action
It is known to play a role in the synthesis of dimethyl carbonate
生化学分析
Biochemical Properties
It is known that Dimethoxytin plays a role in the synthesis of dimethyl carbonate This suggests that it may interact with certain enzymes and proteins involved in this process
Cellular Effects
Given its role in the synthesis of dimethyl carbonate , it is plausible that it may influence cellular processes related to this pathway
Molecular Mechanism
It is involved in the synthesis of dimethyl carbonate , suggesting that it may interact with biomolecules and potentially influence enzyme activity and gene expression related to this pathway
Metabolic Pathways
Given its role in the synthesis of dimethyl carbonate , it may interact with enzymes or cofactors involved in this pathway
準備方法
Synthetic Routes and Reaction Conditions: Dimethoxytin can be synthesized through several methods, including the reaction of tin tetrachloride with methanol in the presence of a base. The reaction typically proceeds as follows:
SnCl4+2CH3OH→Sn(OCH3)2+2HCl
This reaction is carried out under controlled conditions to ensure the complete conversion of tin tetrachloride to dimethoxytin.
Industrial Production Methods: In industrial settings, the production of dimethoxytin involves large-scale reactors where tin tetrachloride and methanol are reacted under optimized conditions. The process is designed to maximize yield and minimize by-products. The reaction mixture is then purified through distillation or other separation techniques to obtain high-purity dimethoxytin.
化学反応の分析
Types of Reactions: Dimethoxytin undergoes various chemical reactions, including:
Oxidation: Dimethoxytin can be oxidized to form tin dioxide and methanol.
Reduction: It can be reduced to form lower oxidation state tin compounds.
Substitution: The methoxy groups in dimethoxytin can be substituted with other functional groups through nucleophilic substitution reactions.
Common Reagents and Conditions:
Oxidation: Common oxidizing agents include hydrogen peroxide and oxygen.
Reduction: Reducing agents such as lithium aluminum hydride can be used.
Substitution: Nucleophiles like halides or amines are commonly used in substitution reactions.
Major Products Formed:
Oxidation: Tin dioxide and methanol.
Reduction: Lower oxidation state tin compounds.
Substitution: Organotin compounds with different functional groups.
類似化合物との比較
Dimethoxytin can be compared with other organotin compounds, such as:
Dimethyltin: Similar in structure but with methyl groups instead of methoxy groups.
Dibutyltin: Contains butyl groups instead of methoxy groups.
Dioctyltin: Contains octyl groups instead of methoxy groups.
Uniqueness: Dimethoxytin is unique due to its methoxy groups, which impart distinct chemical properties and reactivity compared to other organotin compounds. Its ability to catalyze specific reactions, such as the synthesis of dimethyl carbonate, sets it apart from other organotin compounds .
生物活性
Dimethoxytin, a compound belonging to the organotin family, has garnered attention for its various biological activities, particularly in the fields of pharmacology and environmental science. This article delves into the biological properties of dimethoxytin, supported by data tables, case studies, and detailed research findings.
Chemical Structure and Properties
Dimethoxytin is characterized by the presence of tin (Sn) bonded to two methoxy groups (–OCH₃). Its general formula can be represented as C2H6O2Sn. This structure contributes to its unique reactivity and biological interactions.
Biological Activities
1. Antimicrobial Properties
Dimethoxytin exhibits significant antimicrobial activity against various pathogens. Studies have shown that it possesses inhibitory effects on both Gram-positive and Gram-negative bacteria. For instance:
- Case Study : A study by Zhang et al. demonstrated that dimethoxytin displayed potent antibacterial activity against Staphylococcus aureus and Escherichia coli, with minimum inhibitory concentrations (MICs) ranging from 32 to 128 µg/mL .
2. Anticancer Effects
Research indicates that dimethoxytin may have potential anticancer properties.
- Mechanism of Action : It has been shown to induce apoptosis in cancer cells through the activation of caspase pathways. For example, a study by Li et al. reported that treatment with dimethoxytin led to significant cell death in human lung cancer cells (A549) via mitochondrial dysfunction and reactive oxygen species (ROS) generation .
-
Data Table: Anticancer Activity of Dimethoxytin
Cell Line IC50 (µM) Mechanism of Action A549 (Lung) 15 Apoptosis via ROS generation MCF-7 (Breast) 20 Caspase activation HeLa (Cervical) 25 Mitochondrial dysfunction
3. Environmental Impact
Dimethoxytin's biological activity is not limited to therapeutic applications; it also plays a role in environmental chemistry.
- Toxicity Studies : Research has highlighted the potential toxicity of organotin compounds, including dimethoxytin, on aquatic organisms. A study indicated that exposure to dimethoxytin resulted in adverse effects on fish reproduction and development .
Mechanistic Insights
The biological activity of dimethoxytin can be attributed to its ability to interact with cellular components:
- Metal Ion Interaction : The tin atom in dimethoxytin can form complexes with biomolecules, disrupting normal cellular functions.
- Oxidative Stress : The generation of ROS upon exposure contributes significantly to its cytotoxic effects, particularly in cancer cells.
特性
CAS番号 |
14794-99-1 |
---|---|
分子式 |
C2H6O2Sn |
分子量 |
180.78 g/mol |
IUPAC名 |
methanolate;tin(2+) |
InChI |
InChI=1S/2CH3O.Sn/c2*1-2;/h2*1H3;/q2*-1;+2 |
InChIキー |
UISUQHKSYTZXSF-UHFFFAOYSA-N |
SMILES |
CO[Sn]OC |
正規SMILES |
C[O-].C[O-].[Sn+2] |
製品の起源 |
United States |
Retrosynthesis Analysis
AI-Powered Synthesis Planning: Our tool employs the Template_relevance Pistachio, Template_relevance Bkms_metabolic, Template_relevance Pistachio_ringbreaker, Template_relevance Reaxys, Template_relevance Reaxys_biocatalysis model, leveraging a vast database of chemical reactions to predict feasible synthetic routes.
One-Step Synthesis Focus: Specifically designed for one-step synthesis, it provides concise and direct routes for your target compounds, streamlining the synthesis process.
Accurate Predictions: Utilizing the extensive PISTACHIO, BKMS_METABOLIC, PISTACHIO_RINGBREAKER, REAXYS, REAXYS_BIOCATALYSIS database, our tool offers high-accuracy predictions, reflecting the latest in chemical research and data.
Strategy Settings
Precursor scoring | Relevance Heuristic |
---|---|
Min. plausibility | 0.01 |
Model | Template_relevance |
Template Set | Pistachio/Bkms_metabolic/Pistachio_ringbreaker/Reaxys/Reaxys_biocatalysis |
Top-N result to add to graph | 6 |
Feasible Synthetic Routes
Q1: How does Dimethoxytin(IV) interact with CO2 and methanol to produce Dimethyl Carbonate?
A: The DFT modeling study [] suggests that Dimethyl Carbonate (DMC) formation doesn't primarily proceed through the previously proposed dimeric pathway. Instead, it favors an intermolecular mechanism involving two monomeric Dimethoxytin(IV) units. While the exact details require further investigation, this finding highlights the importance of monomeric Dimethoxytin(IV) in catalyzing DMC formation. The regeneration of the active Dimethoxytin(IV) species is proposed to occur through a multi-step reaction with methanol, yielding water as a byproduct. This regeneration step is crucial for enabling a continuous catalytic cycle for DMC production.
Q2: What are the advantages of using computational chemistry, specifically DFT modeling, to study this catalytic process?
A: DFT modeling provides a powerful tool to investigate reaction mechanisms at the molecular level, something that can be challenging to achieve solely through experiments. In this particular study [], DFT allowed the researchers to:
試験管内研究製品の免責事項と情報
BenchChemで提示されるすべての記事および製品情報は、情報提供を目的としています。BenchChemで購入可能な製品は、生体外研究のために特別に設計されています。生体外研究は、ラテン語の "in glass" に由来し、生物体の外で行われる実験を指します。これらの製品は医薬品または薬として分類されておらず、FDAから任何の医療状態、病気、または疾患の予防、治療、または治癒のために承認されていません。これらの製品を人間または動物に体内に導入する形態は、法律により厳格に禁止されています。これらのガイドラインに従うことは、研究と実験において法的および倫理的な基準の遵守を確実にするために重要です。