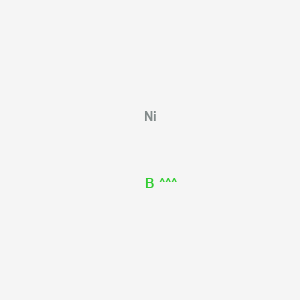
Nickel boride (NiB)
説明
Nickel boride is a material composed chiefly of the elements nickel and boron . It is widely used as a catalyst in organic chemistry . The approximate chemical composition is Ni2.5B . It is typically prepared by reacting a salt of nickel with sodium borohydride . Nickel boride is an efficient catalyst and reducing agent . It is used as a heterogeneous hydrogenation catalyst .
Synthesis Analysis
Nickel boride catalysts are typically prepared by reacting a salt of nickel with sodium borohydride . The P−1 catalyst can be generated by reacting a nickel (II) salt, such as sulfate, chloride, nitrate, or acetate, and sodium borohydride in alkaline aqueous solutions . The product precipitates as a fine, black granular powder . The chemistry is very similar to that of electroless nickel-boron plating .
Molecular Structure Analysis
Nickel boride is an intermetallic compound composed of nickel and boron atoms . It is a hard, brittle material with a metallic luster .
Chemical Reactions Analysis
Nickel boride is an efficient catalyst for methanol electrooxidation into formate with a Faradaic efficiency of nearly 100% . High concentrations of methanol inhibit the phase transition of the electrocatalyst to high-valent electro-oxidation products .
科学的研究の応用
Electrocatalysis
Nickel borides are a large family of inorganic solids with rich bonding schemes, and huge compositional and structural diversity. They possess high flexibility to modulate the local electronic structures and surface adsorption properties, providing great opportunities for the development of advanced catalysts with superior activity and stability .
Hydrogen and Oxygen Evolution Reactions
Nickel borides could be a potential catalyst for a broad range of applications such as hydrogenations, electrochemical hydrogen, and oxygen evolution reactions under challenging conditions (such as high pH or high temperatures) .
Water Splitting
Nickel boride/borate amorphous/amorphous heterostructures have been engineered for electrocatalytic water splitting . The heterostructures with the highest nickel content exhibited the highest values of measured current and lowest values of measured resistance .
Energy Storage
Nickel boride has shown superior electrochemical performance in the field of energy storage . However, particle agglomeration and poor conductivity have limited its wider application .
Magnetic Performance
Nickel boride/borate amorphous/amorphous heterostructures have also been studied for their magnetic performance . The heterostructure with the highest nickel content demonstrated better magnetic performance when compared to the other two heterostructures .
Interfacial Engineering
The electron deficient state of B in Ni (BO2)2 amorphous shell will make it easy to accept extra electrons, enhancing the adsorption of OH– at the shell surface. Moreover, Ni (BO2)2 makes strong adhesion between NixB (or β-Ni (OH)2) and graphene and protects the core structure in a stable state, extending the cycle life .
作用機序
Target of Action
Nickel boride (NiB) is a compound composed chiefly of the elements nickel and boron . It is widely used as a catalyst in organic chemistry . The primary targets of NiB are various chemical reactions, such as the hydrogenation of different compounds .
Mode of Action
NiB interacts with its targets through catalytic processes. In the pioneering work of Paul et al., NiB containing small quantities of active promoters (e.g., Mo, W, Cr) were discovered to be more active for the hydrogenation reaction than RANEY® nickel . The compound’s interaction with its targets results in enhanced catalytic activity and stability .
Biochemical Pathways
NiB affects the hydrogenation pathway in various chemical reactions . The compound’s role in these reactions is to accelerate the process, making it more efficient. The downstream effects of this acceleration can include faster reaction times and higher yields of the desired products .
Pharmacokinetics
It’s worth noting that the compound’s catalytic activity can be influenced by the specific preparation method .
Result of Action
The molecular and cellular effects of NiB’s action primarily involve the acceleration of hydrogenation reactions . This can result in the more efficient production of desired products in these reactions .
Action Environment
The action, efficacy, and stability of NiB can be influenced by various environmental factors. For example, high concentrations of methanol inhibit the phase transition of the electrocatalyst to high-valent electro-oxidation products .
将来の方向性
特性
IUPAC Name |
boranylidynenickel | |
---|---|---|
Source | PubChem | |
URL | https://pubchem.ncbi.nlm.nih.gov | |
Description | Data deposited in or computed by PubChem | |
InChI |
InChI=1S/B.Ni | |
Source | PubChem | |
URL | https://pubchem.ncbi.nlm.nih.gov | |
Description | Data deposited in or computed by PubChem | |
InChI Key |
QDWJUBJKEHXSMT-UHFFFAOYSA-N | |
Source | PubChem | |
URL | https://pubchem.ncbi.nlm.nih.gov | |
Description | Data deposited in or computed by PubChem | |
Canonical SMILES |
B#[Ni] | |
Source | PubChem | |
URL | https://pubchem.ncbi.nlm.nih.gov | |
Description | Data deposited in or computed by PubChem | |
Molecular Formula |
BNi | |
Source | PubChem | |
URL | https://pubchem.ncbi.nlm.nih.gov | |
Description | Data deposited in or computed by PubChem | |
DSSTOX Substance ID |
DTXSID4065155 | |
Record name | Nickel boride (NiB) | |
Source | EPA DSSTox | |
URL | https://comptox.epa.gov/dashboard/DTXSID4065155 | |
Description | DSSTox provides a high quality public chemistry resource for supporting improved predictive toxicology. | |
Molecular Weight |
69.51 g/mol | |
Source | PubChem | |
URL | https://pubchem.ncbi.nlm.nih.gov | |
Description | Data deposited in or computed by PubChem | |
Product Name |
Nickel boride (NiB) | |
CAS RN |
12007-02-2, 11099-25-5, 12007-00-0 | |
Record name | Nickel boride (Ni3B) | |
Source | CAS Common Chemistry | |
URL | https://commonchemistry.cas.org/detail?cas_rn=12007-02-2 | |
Description | CAS Common Chemistry is an open community resource for accessing chemical information. Nearly 500,000 chemical substances from CAS REGISTRY cover areas of community interest, including common and frequently regulated chemicals, and those relevant to high school and undergraduate chemistry classes. This chemical information, curated by our expert scientists, is provided in alignment with our mission as a division of the American Chemical Society. | |
Explanation | The data from CAS Common Chemistry is provided under a CC-BY-NC 4.0 license, unless otherwise stated. | |
Record name | Boron alloy, nonbase, B,Ni | |
Source | CAS Common Chemistry | |
URL | https://commonchemistry.cas.org/detail?cas_rn=11099-25-5 | |
Description | CAS Common Chemistry is an open community resource for accessing chemical information. Nearly 500,000 chemical substances from CAS REGISTRY cover areas of community interest, including common and frequently regulated chemicals, and those relevant to high school and undergraduate chemistry classes. This chemical information, curated by our expert scientists, is provided in alignment with our mission as a division of the American Chemical Society. | |
Explanation | The data from CAS Common Chemistry is provided under a CC-BY-NC 4.0 license, unless otherwise stated. | |
Record name | Nickel boride (NiB) | |
Source | CAS Common Chemistry | |
URL | https://commonchemistry.cas.org/detail?cas_rn=12007-00-0 | |
Description | CAS Common Chemistry is an open community resource for accessing chemical information. Nearly 500,000 chemical substances from CAS REGISTRY cover areas of community interest, including common and frequently regulated chemicals, and those relevant to high school and undergraduate chemistry classes. This chemical information, curated by our expert scientists, is provided in alignment with our mission as a division of the American Chemical Society. | |
Explanation | The data from CAS Common Chemistry is provided under a CC-BY-NC 4.0 license, unless otherwise stated. | |
Record name | Nickel boride (NiB) | |
Source | ChemIDplus | |
URL | https://pubchem.ncbi.nlm.nih.gov/substance/?source=chemidplus&sourceid=0012007000 | |
Description | ChemIDplus is a free, web search system that provides access to the structure and nomenclature authority files used for the identification of chemical substances cited in National Library of Medicine (NLM) databases, including the TOXNET system. | |
Record name | Nickel boride (NiB) | |
Source | EPA Chemicals under the TSCA | |
URL | https://www.epa.gov/chemicals-under-tsca | |
Description | EPA Chemicals under the Toxic Substances Control Act (TSCA) collection contains information on chemicals and their regulations under TSCA, including non-confidential content from the TSCA Chemical Substance Inventory and Chemical Data Reporting. | |
Record name | Nickel boride (NiB) | |
Source | EPA DSSTox | |
URL | https://comptox.epa.gov/dashboard/DTXSID4065155 | |
Description | DSSTox provides a high quality public chemistry resource for supporting improved predictive toxicology. | |
Record name | Nickel boride | |
Source | European Chemicals Agency (ECHA) | |
URL | https://echa.europa.eu/substance-information/-/substanceinfo/100.031.344 | |
Description | The European Chemicals Agency (ECHA) is an agency of the European Union which is the driving force among regulatory authorities in implementing the EU's groundbreaking chemicals legislation for the benefit of human health and the environment as well as for innovation and competitiveness. | |
Explanation | Use of the information, documents and data from the ECHA website is subject to the terms and conditions of this Legal Notice, and subject to other binding limitations provided for under applicable law, the information, documents and data made available on the ECHA website may be reproduced, distributed and/or used, totally or in part, for non-commercial purposes provided that ECHA is acknowledged as the source: "Source: European Chemicals Agency, http://echa.europa.eu/". Such acknowledgement must be included in each copy of the material. ECHA permits and encourages organisations and individuals to create links to the ECHA website under the following cumulative conditions: Links can only be made to webpages that provide a link to the Legal Notice page. | |
試験管内研究製品の免責事項と情報
BenchChemで提示されるすべての記事および製品情報は、情報提供を目的としています。BenchChemで購入可能な製品は、生体外研究のために特別に設計されています。生体外研究は、ラテン語の "in glass" に由来し、生物体の外で行われる実験を指します。これらの製品は医薬品または薬として分類されておらず、FDAから任何の医療状態、病気、または疾患の予防、治療、または治癒のために承認されていません。これらの製品を人間または動物に体内に導入する形態は、法律により厳格に禁止されています。これらのガイドラインに従うことは、研究と実験において法的および倫理的な基準の遵守を確実にするために重要です。