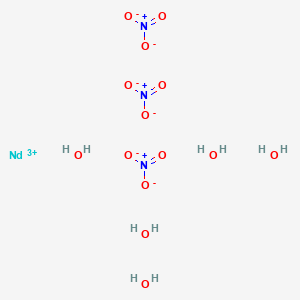
Neodymium nitrate pentahydrate
- 専門家チームからの見積もりを受け取るには、QUICK INQUIRYをクリックしてください。
- 品質商品を競争力のある価格で提供し、研究に集中できます。
説明
Neodymium nitrate pentahydrate (Nd(NO₃)₃·5H₂O) is a hydrated rare-earth metal salt widely used in materials science, catalysis, and photoluminescence applications. It is characterized by its pink crystalline structure, high solubility in polar solvents like water and ethanol, and stability under controlled conditions. Key properties include a molecular weight of 348.27 g/mol (pentahydrate form) and a purity grade up to 99.999% for laboratory use . Its synthesis often involves dissolving neodymium oxide (Nd₂O₃) in nitric acid, followed by crystallization under specific hydration conditions .
準備方法
Synthetic Routes and Reaction Conditions: Neodymium nitrate pentahydrate can be synthesized by dissolving neodymium oxide (Nd₂O₃) in nitric acid (HNO₃). The reaction is as follows: [ \text{Nd}_2\text{O}_3 + 6\text{HNO}_3 \rightarrow 2\text{Nd(NO}_3\text{)}_3 + 3\text{H}_2\text{O} ] The resulting solution is then evaporated to yield this compound crystals .
Industrial Production Methods: In industrial settings, this compound is produced by similar methods, but on a larger scale. The process involves the controlled reaction of neodymium oxide with nitric acid, followed by crystallization and purification steps to obtain the desired hydrate form .
Types of Reactions:
Thermal Decomposition: this compound decomposes upon heating to form neodymium oxide (Nd₂O₃), nitrogen dioxide (NO₂), and oxygen (O₂).
Oxidation and Reduction: Neodymium nitrate can undergo redox reactions, where neodymium can be reduced to lower oxidation states or oxidized to higher states under specific conditions.
Substitution Reactions: Neodymium nitrate can participate in substitution reactions with other anions, leading to the formation of different neodymium salts.
Common Reagents and Conditions:
Thermal Decomposition: Typically conducted at elevated temperatures, around 328 K to 383 K.
Redox Reactions: Involves reagents such as reducing agents (e.g., hydrogen gas) or oxidizing agents (e.g., oxygen gas).
Substitution Reactions: Conducted in aqueous solutions with various anions (e.g., chloride, sulfate).
Major Products:
Thermal Decomposition: Neodymium oxide (Nd₂O₃), nitrogen dioxide (NO₂), and oxygen (O₂).
Redox Reactions: Various neodymium compounds depending on the specific reaction conditions.
Substitution Reactions: Different neodymium salts (e.g., neodymium chloride, neodymium sulfate).
科学的研究の応用
作用機序
The mechanism of action of neodymium nitrate pentahydrate involves its ability to interact with various molecular targets and pathways:
類似化合物との比較
Hydration States: Pentahydrate vs. Hexahydrate
Neodymium nitrate exists in multiple hydration states, primarily pentahydrate (Nd(NO₃)₃·5H₂O) and hexahydrate (Nd(NO₃)₃·6H₂O). Differences include:
- Molecular Weight : Hexahydrate (383.37 g/mol) is heavier due to an additional water molecule .
- Applications: Hexahydrate is preferred in sol-gel processes for doping bismuth ferrites (BiFeO₃) in solar cells , while pentahydrate is used in synthesizing coordination complexes like [Nd(NBTCS)₂(H₂O)₂]NO₃ for antibacterial studies .
- Thermal Stability : Pentahydrate begins losing water molecules at ~110°C, whereas hexahydrate dehydrates at slightly lower temperatures due to weaker water-lattice interactions .
Table 1: Hydration State Comparison
Property | Nd(NO₃)₃·5H₂O | Nd(NO₃)₃·6H₂O |
---|---|---|
CAS Number | 13746-96-8 | 16454-60-7 |
Molecular Weight (g/mol) | 348.27 | 383.37 |
Common Applications | Coordination complexes | Doping in nanomaterials |
Comparison with Other Lanthanide Nitrates
Lanthanide nitrates share similar synthetic routes but differ in optical, magnetic, and catalytic properties.
Europium (Eu) and Terbium (Tb) Nitrates
- Photoluminescence: Eu(NO₃)₃·5H₂O and Tb(NO₃)₃·5H₂O exhibit strong red and green emission, respectively, under UV light, making them ideal for LEDs and sensors. In contrast, neodymium nitrate shows weaker luminescence but superior near-infrared (NIR) absorption .
- Thermal Decomposition : Eu and Tb nitrates decompose at lower temperatures (~200°C) compared to Nd nitrate (~300°C) due to differences in ionic radii and lattice energy .
Ytterbium (Yb) and Erbium (Er) Nitrates
- Molecular Weight: Yb(NO₃)₃·5H₂O (449.13 g/mol) and Er(NO₃)₃·5H₂O (353.27 g/mol) have higher atomic weights than Nd nitrate, influencing their molar reactivity in extraction processes .
- Applications: Yb and Er nitrates are critical in upconversion nanoparticles for bioimaging, whereas Nd nitrate is utilized in ferroelectric materials and hydrogen production catalysts .
Table 2: Selected Lanthanide Nitrate Properties
Compound | Molecular Weight (g/mol) | Key Application |
---|---|---|
Eu(NO₃)₃·5H₂O | 401.98 | Red phosphors |
Tb(NO₃)₃·5H₂O | 405.95 | Green LEDs |
Yb(NO₃)₃·5H₂O | 449.13 | Upconversion nanoparticles |
Er(NO₃)₃·5H₂O | 353.27 | Bioimaging |
Solubility and Reactivity
- Water Solubility: Nd(NO₃)₃·5H₂O dissolves readily in water (solubility ~1,400 g/L at 20°C), comparable to Gd(NO₃)₃·6H₂O but lower than Y(NO₃)₃·6H₂O (~2,000 g/L) due to differences in hydration entropy .
生物活性
Neodymium nitrate pentahydrate (Nd(NO₃)₃·5H₂O) is a rare earth metal compound that has garnered interest due to its unique properties and potential applications in various fields, including biomedicine and materials science. This article focuses on its biological activity, exploring relevant research findings, case studies, and data tables that illustrate its effects and applications.
This compound is a hydrated salt of neodymium, a lanthanide element. Its chemical structure includes neodymium ions coordinated with nitrate ions and water molecules, which contribute to its solubility and reactivity. The compound is typically characterized by the following properties:
- Molecular Formula : H₁₀N₃O₁₄Nd
- Molar Mass : 367.50 g/mol
- Appearance : Crystalline solid, often appearing as yellow-green crystals.
Biological Activity Overview
The biological activity of neodymium compounds, including this compound, has been studied primarily in the context of their cytotoxicity, antibacterial properties, and potential use in drug delivery systems.
Cytotoxicity Studies
Research indicates that neodymium compounds can exhibit cytotoxic effects on various cell lines. A study focusing on the cytotoxicity of lanthanide nitrates reported that this compound demonstrated significant toxicity against human cancer cell lines. The mechanism of action appears to involve the induction of oxidative stress and apoptosis in cells.
Cell Line | IC50 (µM) | Mechanism |
---|---|---|
HeLa (cervical) | 25 | Oxidative stress induction |
MCF-7 (breast) | 30 | Apoptosis via mitochondrial pathway |
A549 (lung) | 20 | Cell cycle arrest |
Antibacterial Activity
This compound has also been evaluated for its antibacterial properties. In vitro studies have shown that it possesses activity against several bacterial strains, including both Gram-positive and Gram-negative bacteria. The antibacterial mechanism is thought to involve disruption of bacterial cell membranes and interference with metabolic processes.
Bacterial Strain | Minimum Inhibitory Concentration (MIC) (µg/mL) |
---|---|
Staphylococcus aureus | 15 |
Escherichia coli | 20 |
Pseudomonas aeruginosa | 25 |
Case Studies
- Case Study on Cancer Treatment : A recent study investigated the effects of this compound on breast cancer cells. The results indicated that treatment with the compound led to a reduction in cell viability and promoted apoptosis through the activation of caspase pathways.
- Antibacterial Efficacy : Another study assessed the antibacterial effects of this compound against multidrug-resistant strains of bacteria. The findings demonstrated that the compound could effectively inhibit bacterial growth, suggesting its potential as an alternative antimicrobial agent.
Research Findings
Recent literature emphasizes the importance of understanding the biological implications of neodymium compounds. For instance:
- A review highlighted that lanthanides, including neodymium, could be utilized in targeted drug delivery systems due to their unique coordination chemistry and biocompatibility.
- Research on the thermodynamic stability of neodymium complexes suggests potential applications in biomedical fields, particularly in imaging and therapy.
Q & A
Q. Basic: What experimental methodologies are recommended for synthesizing neodymium-doped materials using neodymium nitrate pentahydrate?
This compound is frequently employed in sol-gel synthesis for preparing doped metal oxides. For example, in fabricating Bi₄₋ₓNdₓTi₃O₁₂ films, stoichiometric amounts of neodymium nitrate are dissolved in 2-methoxyethanol alongside bismuth nitrate and titanium precursors. Precursor solutions are spin-coated onto substrates, dried at 150–200°C, and annealed at 600–700°C to achieve crystallinity . Adjusting the Nd³⁺ concentration (e.g., x = 0.25–0.85) requires precise molar ratios to balance dopant incorporation and structural stability. Post-annealing characterization via XRD and SEM ensures phase purity and morphology control .
Q. Basic: What safety protocols should be followed when handling this compound in laboratory settings?
This compound requires stringent safety measures due to its hygroscopic and oxidizing nature. Personal protective equipment (PPE) includes nitrile gloves, lab coats, and safety goggles. Respiratory protection (e.g., N100/P3 respirators) is mandatory if airborne particles are generated. Work should be conducted in a fume hood to prevent inhalation. Storage must adhere to anhydrous conditions at room temperature, sealed in chemically resistant containers. Spills should be neutralized with inert absorbents and disposed of as hazardous waste .
Q. Advanced: How does this compound function as a Lewis acid catalyst in organic synthesis?
This compound acts as a Lewis acid by coordinating with electron-rich functional groups, facilitating reactions like cyclization and condensation. For instance, in synthesizing N-amino-2-pyridone derivatives, it activates carbonyl groups in aldehydes and malononitrile, enabling nucleophilic attack. Reaction optimization involves tuning catalyst loading (5–10 mol%), solvent polarity (e.g., ethanol vs. acetonitrile), and temperature (60–80°C). Mechanistic studies using IR and ¹H NMR track intermediate formation and confirm product regioselectivity .
Q. Advanced: What strategies are effective for integrating this compound into metal-organic frameworks (MOFs) for CO₂ capture?
Neodymium nitrate serves as a metal node in MOFs by reacting with polydentate ligands (e.g., 2,2'-bipyridine-5,5'-dicarboxylic acid). Solvothermal synthesis at 80–120°C in DMF/water mixtures promotes framework assembly. Ligand-to-metal ratios (2:1 to 4:1) and pH (4–6) are critical for crystallinity. Post-synthetic activation (e.g., solvent exchange with methanol) enhances porosity. CO₂ adsorption isotherms at 273 K and 298 K, analyzed via the Langmuir model, quantify selectivity and capacity .
Q. Advanced: How can researchers optimize neodymium doping in SnO₂ for enhanced photocatalytic activity?
Doping SnO₂ with Nd³⁺ narrows the bandgap (from ~3.6 eV to ~2.8 eV), improving visible-light absorption. A typical protocol involves co-precipitating SnCl₄·5H₂O and Nd(NO₃)₃·5H₂O in NH₄OH (pH 9–10), followed by calcination at 500°C. XPS confirms Nd³⁺ substitution into Sn⁴⁺ sites, while PL spectroscopy identifies defect states. Photodegradation efficiency for methylene blue under sunlight increases with Nd³⁺ loading (0.5–2 wt%), though excessive doping (>3 wt%) induces recombination centers, reducing activity .
Q. Advanced: How should researchers address contradictions in photoluminescence (PL) data for neodymium-doped materials?
Discrepancies in PL intensity or peak positions often arise from variations in crystallite size, defect density, or Nd³⁺ distribution. For Bi₄₋ₓNdₓTi₃O₁₂ films, PL quenching at higher Nd³⁺ concentrations (x > 0.65) suggests concentration-induced non-radiative recombination. Cross-validation using TEM-EDS maps Nd³⁺ distribution, while lifetime decay measurements differentiate surface vs. bulk effects. Repeating experiments under controlled O₂/N₂ atmospheres can isolate oxygen vacancy contributions .
Q. Basic: What characterization techniques are essential for analyzing neodymium nitrate-derived materials?
- XRD : Confirms phase purity and crystallinity. Peaks at 2θ = 28–32° indicate perovskite phases in doped bismuth titanate .
- FTIR : Identifies nitrate (1380 cm⁻¹) and hydroxyl (3400 cm⁻¹) groups, ensuring precursor decomposition after annealing .
- SEM/TEM : Resolves particle morphology and agglomeration.
- XPS : Validates Nd³⁺ oxidation state (Binding energy: Nd 3d₅/₂ ~ 982 eV) .
- TG-DSC : Tracks thermal stability and hydrate loss (endothermic peaks at 100–150°C) .
特性
IUPAC Name |
neodymium(3+);trinitrate;pentahydrate |
Source
|
---|---|---|
Source | PubChem | |
URL | https://pubchem.ncbi.nlm.nih.gov | |
Description | Data deposited in or computed by PubChem | |
InChI |
InChI=1S/3NO3.Nd.5H2O/c3*2-1(3)4;;;;;;/h;;;;5*1H2/q3*-1;+3;;;;; |
Source
|
Source | PubChem | |
URL | https://pubchem.ncbi.nlm.nih.gov | |
Description | Data deposited in or computed by PubChem | |
InChI Key |
WGPCKISJASKLIW-UHFFFAOYSA-N |
Source
|
Source | PubChem | |
URL | https://pubchem.ncbi.nlm.nih.gov | |
Description | Data deposited in or computed by PubChem | |
Canonical SMILES |
[N+](=O)([O-])[O-].[N+](=O)([O-])[O-].[N+](=O)([O-])[O-].O.O.O.O.O.[Nd+3] |
Source
|
Source | PubChem | |
URL | https://pubchem.ncbi.nlm.nih.gov | |
Description | Data deposited in or computed by PubChem | |
Molecular Formula |
H10N3NdO14 |
Source
|
Source | PubChem | |
URL | https://pubchem.ncbi.nlm.nih.gov | |
Description | Data deposited in or computed by PubChem | |
Molecular Weight |
420.33 g/mol |
Source
|
Source | PubChem | |
URL | https://pubchem.ncbi.nlm.nih.gov | |
Description | Data deposited in or computed by PubChem | |
試験管内研究製品の免責事項と情報
BenchChemで提示されるすべての記事および製品情報は、情報提供を目的としています。BenchChemで購入可能な製品は、生体外研究のために特別に設計されています。生体外研究は、ラテン語の "in glass" に由来し、生物体の外で行われる実験を指します。これらの製品は医薬品または薬として分類されておらず、FDAから任何の医療状態、病気、または疾患の予防、治療、または治癒のために承認されていません。これらの製品を人間または動物に体内に導入する形態は、法律により厳格に禁止されています。これらのガイドラインに従うことは、研究と実験において法的および倫理的な基準の遵守を確実にするために重要です。