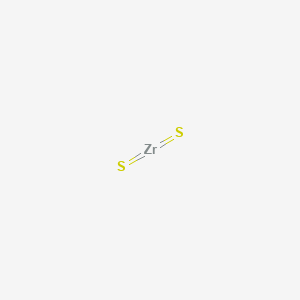
Zirconium sulfide (ZrS2)
説明
Zirconium (IV) sulfide, also known as Zirconium disulfide, is an inorganic compound with the formula ZrS2 . It is a violet-brown solid that adopts a layered structure similar to that of cadmium iodide . It is a kind of red-brown crystal with a metallic luster .
Synthesis Analysis
Zirconium disulfide (ZrS2) is prepared by heating sulfur and zirconium metal . A diffusion method has been used to synthesize ZrS2 from elemental zirconium and sulfur, which allows control over the morphology and phases of zirconium sulfides by tuning the reaction temperature and sulfur vapor pressure .Molecular Structure Analysis
Zirconium disulfide adopts a layered structure similar to that of cadmium iodide . The structure is two-dimensional and consists of one ZrS2 sheet oriented in the (0, 0, 1) direction . Zr4+ is bonded to six equivalent S2- atoms to form edge-sharing ZrS6 octahedra .Chemical Reactions Analysis
Zirconium disulfide can undergo oxidation, which is a major issue for its long-term stability . The oxidation rate is highly dependent on the initial adsorption of oxygen molecules on the surface .Physical And Chemical Properties Analysis
Zirconium disulfide has a molar mass of 155.356 g/mol and a density of 3.82 g/cm3 . It is insoluble in water . The calculated bulk crystalline density is 3.31 g/cm3 . It has a band gap of 1.042 eV .科学的研究の応用
Zirconium Sulfide in Photocatalysis
Zirconium sulfide (ZrS2): has been explored for its photocatalytic properties, particularly in the degradation of textile dyes . The compound’s ability to operate under visible light makes it an attractive option for environmental cleanup efforts. Its high photocatalytic degradation efficiency is mainly due to the suppression of charge carrier recombination, which is crucial in the breakdown of organic pollutants.
Zirconium Sulfide in Biomedical Applications
In the biomedical field, ZrS2 is part of the zirconium-based metal–organic frameworks (MOFs) that have been investigated for drug delivery, anti-cancer activity, bio-sensing, and imaging . These frameworks are known for their stability, low cytotoxicity, and high drug-loading capacity, making them suitable for various therapeutic and diagnostic applications.
Zirconium Sulfide in Energy Storage
Zirconium sulfide: is also being studied for its potential use in energy storage systems. Research has shown that zirconia-based carbon nanofibers, which include ZrS2 , can be used in supercapacitors, offering high specific capacitance and excellent cycling stability . This could lead to more efficient energy storage solutions.
Zirconium Sulfide in Electronics
The electronic properties of ZrS2 make it a candidate for use in electronics, particularly in thin films that offer exceptional electronic properties . These films can be used in photovoltaics and light-emitting diodes (LEDs), combining strong light absorption with good charge transport.
Zirconium Sulfide in Environmental Science
Zirconium sulfide: plays a role in environmental science as a component in nanocomposites used for the degradation of pollutants . Its effectiveness in removing dyes from water bodies highlights its potential in addressing water pollution challenges.
Zirconium Sulfide in Material Science
In material science, ZrS2 is recognized for its unique crystalline structure and properties, such as a stable band gap and high density, which are beneficial in various applications, including as substrates and in the development of new materials .
Zirconium Sulfide in Industrial Uses
Industrially, ZrS2 is part of the broader category of zirconium compounds used in ignition charges, automotive airbag inflators, pyrotechnics, and military applications such as incendiary charges . Its properties are leveraged in contexts where durability and resistance to extreme conditions are required.
作用機序
Target of Action
Zirconium sulfide (ZrS2) is an inorganic compound that adopts a layered structure It’s known that zrs2 interacts with various elements and compounds during its formation and application .
Mode of Action
The mode of action of ZrS2 is largely dependent on its physical and chemical properties. It is a violet-brown solid that adopts a layered structure similar to that of cadmium iodide . ZrS2 is prepared by heating sulfur and zirconium metal . Zirconium (Zr4+) is bonded to six equivalent S2- atoms to form edge-sharing ZrS6 octahedra . The interaction of ZrS2 with its targets results in changes that are dependent on the specific application and environment.
Biochemical Pathways
Zirconium-based materials have been investigated for various bio-related applications such as drug delivery, anti-cancer activity, bio-sensing, and imaging . The specific pathways and downstream effects would depend on the specific application and the biological system in which ZrS2 is utilized.
Pharmacokinetics
The pharmacokinetics of nanoparticles, which zrs2 can be categorized under, is believed to be controlled by a complex array of interrelated physicochemical and biological factors . The impact on bioavailability would depend on these factors and the specific biological system in which ZrS2 is utilized.
Result of Action
Zirconium-based materials have been investigated for various bio-related applications such as drug delivery, anti-cancer activity, bio-sensing, and imaging . The specific results of action would depend on the specific application and the biological system in which ZrS2 is utilized.
Safety and Hazards
将来の方向性
特性
IUPAC Name |
bis(sulfanylidene)zirconium | |
---|---|---|
Source | PubChem | |
URL | https://pubchem.ncbi.nlm.nih.gov | |
Description | Data deposited in or computed by PubChem | |
InChI |
InChI=1S/2S.Zr | |
Source | PubChem | |
URL | https://pubchem.ncbi.nlm.nih.gov | |
Description | Data deposited in or computed by PubChem | |
InChI Key |
WVMYSOZCZHQCSG-UHFFFAOYSA-N | |
Source | PubChem | |
URL | https://pubchem.ncbi.nlm.nih.gov | |
Description | Data deposited in or computed by PubChem | |
Canonical SMILES |
S=[Zr]=S | |
Source | PubChem | |
URL | https://pubchem.ncbi.nlm.nih.gov | |
Description | Data deposited in or computed by PubChem | |
Molecular Formula |
ZrS2, S2Zr | |
Record name | Zirconium(IV) sulfide | |
Source | Wikipedia | |
URL | https://en.wikipedia.org/wiki/Zirconium(IV)_sulfide | |
Description | Chemical information link to Wikipedia. | |
Record name | zirconium sulfide | |
Source | Wikipedia | |
URL | https://en.wikipedia.org/wiki/Dictionary_of_chemical_formulas | |
Description | Chemical information link to Wikipedia. | |
Source | PubChem | |
URL | https://pubchem.ncbi.nlm.nih.gov | |
Description | Data deposited in or computed by PubChem | |
DSSTOX Substance ID |
DTXSID7065205 | |
Record name | Zirconium sulfide (ZrS2) | |
Source | EPA DSSTox | |
URL | https://comptox.epa.gov/dashboard/DTXSID7065205 | |
Description | DSSTox provides a high quality public chemistry resource for supporting improved predictive toxicology. | |
Molecular Weight |
155.36 g/mol | |
Source | PubChem | |
URL | https://pubchem.ncbi.nlm.nih.gov | |
Description | Data deposited in or computed by PubChem | |
Physical Description |
Reddish-brown powder; [MSDSonline] | |
Record name | Zirconium sulfide | |
Source | Haz-Map, Information on Hazardous Chemicals and Occupational Diseases | |
URL | https://haz-map.com/Agents/8496 | |
Description | Haz-Map® is an occupational health database designed for health and safety professionals and for consumers seeking information about the adverse effects of workplace exposures to chemical and biological agents. | |
Explanation | Copyright (c) 2022 Haz-Map(R). All rights reserved. Unless otherwise indicated, all materials from Haz-Map are copyrighted by Haz-Map(R). No part of these materials, either text or image may be used for any purpose other than for personal use. Therefore, reproduction, modification, storage in a retrieval system or retransmission, in any form or by any means, electronic, mechanical or otherwise, for reasons other than personal use, is strictly prohibited without prior written permission. | |
Product Name |
Zirconium sulfide (ZrS2) | |
CAS RN |
12039-15-5 | |
Record name | Zirconium sulfide (ZrS2) | |
Source | CAS Common Chemistry | |
URL | https://commonchemistry.cas.org/detail?cas_rn=12039-15-5 | |
Description | CAS Common Chemistry is an open community resource for accessing chemical information. Nearly 500,000 chemical substances from CAS REGISTRY cover areas of community interest, including common and frequently regulated chemicals, and those relevant to high school and undergraduate chemistry classes. This chemical information, curated by our expert scientists, is provided in alignment with our mission as a division of the American Chemical Society. | |
Explanation | The data from CAS Common Chemistry is provided under a CC-BY-NC 4.0 license, unless otherwise stated. | |
Record name | Zirconium sulfide (ZrS2) | |
Source | ChemIDplus | |
URL | https://pubchem.ncbi.nlm.nih.gov/substance/?source=chemidplus&sourceid=0012039155 | |
Description | ChemIDplus is a free, web search system that provides access to the structure and nomenclature authority files used for the identification of chemical substances cited in National Library of Medicine (NLM) databases, including the TOXNET system. | |
Record name | Zirconium sulfide (ZrS2) | |
Source | EPA Chemicals under the TSCA | |
URL | https://www.epa.gov/chemicals-under-tsca | |
Description | EPA Chemicals under the Toxic Substances Control Act (TSCA) collection contains information on chemicals and their regulations under TSCA, including non-confidential content from the TSCA Chemical Substance Inventory and Chemical Data Reporting. | |
Record name | Zirconium sulfide (ZrS2) | |
Source | EPA DSSTox | |
URL | https://comptox.epa.gov/dashboard/DTXSID7065205 | |
Description | DSSTox provides a high quality public chemistry resource for supporting improved predictive toxicology. | |
Record name | Zirconium disulphide | |
Source | European Chemicals Agency (ECHA) | |
URL | https://echa.europa.eu/substance-information/-/substanceinfo/100.031.701 | |
Description | The European Chemicals Agency (ECHA) is an agency of the European Union which is the driving force among regulatory authorities in implementing the EU's groundbreaking chemicals legislation for the benefit of human health and the environment as well as for innovation and competitiveness. | |
Explanation | Use of the information, documents and data from the ECHA website is subject to the terms and conditions of this Legal Notice, and subject to other binding limitations provided for under applicable law, the information, documents and data made available on the ECHA website may be reproduced, distributed and/or used, totally or in part, for non-commercial purposes provided that ECHA is acknowledged as the source: "Source: European Chemicals Agency, http://echa.europa.eu/". Such acknowledgement must be included in each copy of the material. ECHA permits and encourages organisations and individuals to create links to the ECHA website under the following cumulative conditions: Links can only be made to webpages that provide a link to the Legal Notice page. | |
Q & A
Q1: What is the molecular formula and weight of Zirconium sulfide?
A: The molecular formula of Zirconium sulfide is ZrS2. Its molecular weight is 151.4 g/mol. []
Q2: What are some key spectroscopic data points for ZrS2?
A: While specific spectroscopic data depends on the analysis method, research indicates the presence of Zr-S bonding, observable through techniques like Raman and infrared spectroscopy. [] Additionally, studies on its electronic structure provide valuable insights into its band gap and potential for applications like photovoltaics. [, ]
Q3: Can Zirconium sulfide be incorporated into composites?
A: Yes, adding Zirconium sulfide to Zinc sulfide enhances the composite's fracture toughness through a crack-bridging mechanism. This improvement doesn't compromise strength, even though pure Zirconium sulfide is weaker than Zinc sulfide. []
Q4: Does Zirconium sulfide exhibit catalytic activity?
A: Research suggests the reversible conversion between ZrS3 and ZrS2 through thermal decomposition and sulfurization, indicating potential for applications like H2S splitting for hydrogen recovery. []
Q5: What are the potential applications of Zirconium sulfide in energy conversion?
A: Both BaZrS3 and SrZrS3 have shown promise as solar absorber materials due to their suitable band gaps and strong light absorption in the visible region. [, ]
Q6: Have computational methods been applied to study Zirconium sulfide?
A: Yes, Density Functional Theory (DFT) calculations have been instrumental in understanding the degradation mechanisms of BaZrS3 and comparing them to other perovskite materials. [] First-principles calculations based on screened hybrid DFT have also been used to investigate the structural, elastic, electronic, and optical properties of SrZrS3. []
Q7: What are the challenges in formulating Zirconium sulfide-based materials?
A: While BaZrS3 demonstrates improved moisture stability, challenges remain in achieving efficient high-quality thin film growth for practical applications. [] Controlling grain size during synthesis is crucial, as only films sulfurized at high temperatures (1000°C) exhibit the large grains necessary for optoelectronic devices. []
Q8: Are there specific considerations for recycling and waste management related to Zirconium sulfide?
A8: While specific research on ZrS2 recycling is limited, responsible practices for handling and disposal are essential. Sustainable synthesis methods and exploration of closed-loop processes could minimize environmental impact.
Q9: What tools and resources are important for research on Zirconium sulfide?
A9: Essential resources include access to material characterization techniques like X-ray diffraction, electron microscopy, and spectroscopic methods. Computational tools like DFT software are crucial for theoretical modeling and property prediction. Collaboration between material scientists, chemists, and physicists is vital for advancing the field.
Q10: What are some significant milestones in the research of Zirconium sulfide?
A: The discovery of BaZrS3 as a stable, environmentally friendly chalcogenide perovskite has sparked significant interest in its potential for various applications. Research into its synthesis methods, property tuning through doping, and performance in devices represents significant milestones in the field. [, , ]
試験管内研究製品の免責事項と情報
BenchChemで提示されるすべての記事および製品情報は、情報提供を目的としています。BenchChemで購入可能な製品は、生体外研究のために特別に設計されています。生体外研究は、ラテン語の "in glass" に由来し、生物体の外で行われる実験を指します。これらの製品は医薬品または薬として分類されておらず、FDAから任何の医療状態、病気、または疾患の予防、治療、または治癒のために承認されていません。これらの製品を人間または動物に体内に導入する形態は、法律により厳格に禁止されています。これらのガイドラインに従うことは、研究と実験において法的および倫理的な基準の遵守を確実にするために重要です。