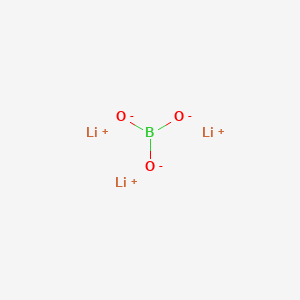
Lithium borate
概要
説明
Lithium borate, also known as lithium tetraborate, is an inorganic compound with the chemical formula Li₂B₄O₇. It is a colorless solid that is widely used in the production of glasses and ceramics. The compound has a polymeric borate backbone structure, with lithium ions bound to four and five oxygen ligands, and boron centers that are trigonal and tetrahedral .
準備方法
Synthetic Routes and Reaction Conditions: Lithium borate can be synthesized through various methods, including high-temperature solid-state reactions and fusion techniques. One common method involves the reaction of lithium carbonate (Li₂CO₃) with boric acid (H₃BO₃) at elevated temperatures. The reaction proceeds as follows: [ \text{Li}_2\text{CO}_3 + 4\text{H}_3\text{BO}_3 \rightarrow \text{Li}_2\text{B}_4\text{O}_7 + 6\text{H}_2\text{O} + \text{CO}_2 ]
Industrial Production Methods: In industrial settings, this compound is often produced using fusion techniques. The sample is mixed with a this compound flux and heated in a platinum crucible until it melts to form a homogeneous liquid. This liquid is then cooled to produce a glassy solid, which can be used for various applications .
化学反応の分析
Electrochemical Reactions in Battery Systems
Lithium borate derivatives enhance high-voltage lithium-ion battery performance:
-
Additive Synthesis:
Lithium alkoxides/phenoxides react with trimethyl borate:
LiOR + B(OCH₃)₃ → LiB(OR)(OCH₃)₃⁻ + CH₃OH
These additives form cathode passivation films, inhibiting Mn dissolution and electrolyte oxidation . -
Coating Effects:
5 wt% Li₂B₄O₇ coating on LiCoO₂ cathodes:
Hydrolysis Pathways and Thermodynamics
Borate-based lithium salts exhibit distinct hydrolysis behaviors:
LiBF₄ Hydrolysis:
LiBF₄ + 2H₂O → LiF(s) + BF₃ + 3HF
ΔG° = -25 kJ/mol (favors LiF precipitation) .
LiBOB Stability:
LiB(C₂O₄)₂ (LiBOB) resists hydrolysis (ΔG° = +74 kJ/mol) due to stable oxalate coordination .
Salt | Hydrolysis Product | ΔG° (kJ/mol) | Dominant Pathway |
---|---|---|---|
LiBF₄ | LiF, HF | -25 | LiF precipitation |
LiODBF | LiF, H₂C₂O₄ | +120 | Oxalate release |
LiBOB | No reaction | +74 | Electrochemically driven |
Reductive Behavior in Solvent Systems
Borate anions demonstrate unique lithium reduction capabilities:
-
Li⁺ Reduction:
[Bipyridine-borate]⁻ + Li⁺ → Li(0) + [Bipyridine-borate]·
Occurs in toluene, producing lithium metal and boron radicals . -
Two-Electron Transfer:
[Borate]⁻ → [Boronium]⁺ + 2e⁻
Applied in CO₂ reduction to CO (FE = 92%) .
High-Temperature Structural Transitions
In this compound glasses/melts, temperature induces coordination changes:
-
Boron Coordination Shift:
BO₃ → BO₄ conversion above Tg (glass transition) in Li-rich compositions . -
Melt Polymerization:
Li₂O Content | Dominant Species | NBO Content | Li⁺ Diffusion Activation Energy |
---|---|---|---|
0–30 mol% | BO₄ | <5% | High (~0.8 eV) |
30–45 mol% | BO₃ + NBOs | 5–15% | Moderate (~0.6 eV) |
>45 mol% | NBOs | >15% | Low (~0.4 eV) |
科学的研究の応用
2.1. Lithium Borate in Lithium-Ion Batteries
This compound salts are gaining attention for their potential use in lithium-ion batteries (LIBs). Their attributes include excellent thermal stability, comparable ionic conductivity, and favorable solid electrolyte interfaces. Recent studies indicate that this compound can enhance the performance of Si/C composite anodes and lithium metal anodes, making them suitable for high-performance batteries .
Case Study: High-Performance Lithium Batteries
- Research Focus : Utilization of this compound salts in high-capacity lithium batteries.
- Findings : this compound improves ionic conductivity and thermal stability, leading to enhanced battery performance under high voltage conditions .
2.2. Optical Applications
This compound is also explored as a nonlinear optical material due to its unique structural properties that allow for second-order nonlinear optical effects. It has been used in developing ultraviolet (UV) and deep-UV coherent light sources .
Material Type | Application |
---|---|
Nonlinear Optical Crystals | UV Lasers |
Birefringent Materials | Light Polarization |
Case Study: Nonlinear Optical Materials
- Research Focus : Development of this compound-based crystals for laser applications.
- Outcome : Successful synthesis of materials that exhibit significant birefringence and noncentrosymmetric structures, ideal for optical devices .
2.3. Dosimetry
This compound serves as a thermoluminescent dosimeter (TLD), particularly when doped with manganese or copper. The compound's ability to measure ionizing radiation makes it valuable in medical and environmental dosimetry.
Dosimeter Type | Sensitivity Level |
---|---|
Li2B4O7:Mn | Standard sensitivity |
Li2B4O7:Cu | Approximately 20x more sensitive |
Case Study: Radiation Dosimetry
- Research Focus : Comparison of sensitivity between different this compound doped materials.
- Findings : Li2B4O7:Cu demonstrated superior sensitivity to gamma rays compared to traditional Li2B4O7:Mn .
Environmental Applications
Lithium borates are being investigated for their role in carbon dioxide capture technologies. Specifically, lithium metaborate (LiBO2) has shown promise as a CO2 adsorbent at elevated temperatures.
Research Insights
- Mechanism : Lithium metaborate reacts with CO2 to form lithium carbonate and other borate compounds, facilitating carbon capture.
- Performance Metrics : High CO2 uptake capacity (11.3 mmol/g at 520 °C) with excellent cyclic renewability .
Analytical Chemistry Applications
This compound fusion is widely used in the preparation of samples for X-ray fluorescence (XRF) and inductively coupled plasma mass spectrometry (ICP-MS). This method allows for the analysis of major and trace elements in silicate rocks.
Technique | Application |
---|---|
XRF | Elemental analysis |
ICP-MS | Trace element detection |
Case Study: Geochemical Analysis
作用機序
The mechanism by which lithium borate exerts its effects involves its interaction with various molecular targets and pathways:
Electrolyte Additive: In lithium-ion batteries, this compound forms a stable interfacial film on the electrodes, enhancing the stability and performance of the battery.
Buffer: In gel electrophoresis, this compound maintains the pH and ionic strength of the buffer solution, ensuring efficient separation of nucleic acids.
Flux: In XRF and ICP analysis, this compound acts as a flux to dissolve samples and produce homogeneous glass discs for accurate analysis .
類似化合物との比較
Lithium Metaborate (LiBO₂): Used in similar applications as lithium borate but has a different structure and melting point.
Lithium Triborate (LiB₃O₅): Known for its non-linear optical properties and used in laser applications.
Lithium Bis(oxalate)borate (LiBOB): Commonly used as an electrolyte additive in lithium-ion batteries.
Uniqueness: this compound is unique due to its polymeric borate backbone structure, which provides excellent thermal stability and ionic conductivity. Its versatility in various applications, from scientific research to industrial production, sets it apart from other borate compounds .
生物活性
Lithium borate, particularly in the form of lithium tetraborate, has garnered attention for its potential biological activities and therapeutic applications. This article delves into the biological effects of this compound, emphasizing its cardioprotective properties, mechanisms of action, and implications for treatment in various medical conditions.
Overview of this compound
This compound is a compound formed from lithium and boron, often utilized in various industrial applications, including glass and ceramics. However, its biological activity has prompted research into its potential therapeutic uses, particularly in cardiology and neuroprotection.
Cardioprotective Effects
Recent studies have highlighted the cardioprotective effects of lithium tetraborate, demonstrating its ability to mitigate damage during ischemia-reperfusion (I/R) injury. This section outlines key findings from relevant research.
- Reduction of Oxidative Stress : Lithium tetraborate has been shown to significantly decrease levels of malondialdehyde (MDA), a marker of oxidative stress, while enhancing antioxidant enzyme activities such as superoxide dismutase (SOD) and glutathione (GSH) in cardiac tissue .
- Anti-inflammatory Properties : The compound reduces inflammatory cytokines such as TNF-α and IL-6, which are typically elevated during I/R injury. This reduction correlates with decreased macrophage activity and overall inflammation in cardiac tissues .
- Anti-apoptotic Effects : Lithium tetraborate treatment leads to a marked decrease in apoptotic markers such as Bax protein levels in cardiac cells, suggesting a protective effect against cell death during ischemic events .
Research Findings and Case Studies
Several studies have documented the biological activity of this compound, particularly focusing on its cardioprotective properties:
Safety and Toxicity Profile
The safety profile of this compound has also been examined, particularly concerning acute toxicity:
- Acute Toxicity : Studies indicate that lithium borates exhibit low acute toxicity levels in animal models, with LD50 values exceeding 2000 mg/kg for both boric acid and lithium carbonate .
- Respiratory Effects : Inhalation studies have reported respiratory irritation but not severe lung function impairment, suggesting that while caution is warranted, the risks may be manageable under controlled conditions .
Future Directions
Despite promising findings regarding the biological activities of this compound, further research is necessary to fully elucidate its mechanisms and potential clinical applications:
- Clinical Trials : There is a need for well-designed clinical trials to assess the efficacy of lithium tetraborate in human populations suffering from cardiovascular diseases.
- Mechanistic Studies : Additional studies exploring the molecular pathways influenced by this compound could provide insights into its broader therapeutic potential beyond cardioprotection.
特性
IUPAC Name |
trilithium;borate | |
---|---|---|
Source | PubChem | |
URL | https://pubchem.ncbi.nlm.nih.gov | |
Description | Data deposited in or computed by PubChem | |
InChI |
InChI=1S/BO3.3Li/c2-1(3)4;;;/q-3;3*+1 | |
Source | PubChem | |
URL | https://pubchem.ncbi.nlm.nih.gov | |
Description | Data deposited in or computed by PubChem | |
InChI Key |
RIUWBIIVUYSTCN-UHFFFAOYSA-N | |
Source | PubChem | |
URL | https://pubchem.ncbi.nlm.nih.gov | |
Description | Data deposited in or computed by PubChem | |
Canonical SMILES |
[Li+].[Li+].[Li+].B([O-])([O-])[O-] | |
Source | PubChem | |
URL | https://pubchem.ncbi.nlm.nih.gov | |
Description | Data deposited in or computed by PubChem | |
Molecular Formula |
BLi3O3 | |
Source | PubChem | |
URL | https://pubchem.ncbi.nlm.nih.gov | |
Description | Data deposited in or computed by PubChem | |
DSSTOX Substance ID |
DTXSID40926674 | |
Record name | Trilithium borate | |
Source | EPA DSSTox | |
URL | https://comptox.epa.gov/dashboard/DTXSID40926674 | |
Description | DSSTox provides a high quality public chemistry resource for supporting improved predictive toxicology. | |
Molecular Weight |
79.7 g/mol | |
Source | PubChem | |
URL | https://pubchem.ncbi.nlm.nih.gov | |
Description | Data deposited in or computed by PubChem | |
CAS No. |
12676-27-6, 1303-94-2 | |
Record name | Boric acid, lithium salt | |
Source | ChemIDplus | |
URL | https://pubchem.ncbi.nlm.nih.gov/substance/?source=chemidplus&sourceid=0012676276 | |
Description | ChemIDplus is a free, web search system that provides access to the structure and nomenclature authority files used for the identification of chemical substances cited in National Library of Medicine (NLM) databases, including the TOXNET system. | |
Record name | Trilithium borate | |
Source | EPA DSSTox | |
URL | https://comptox.epa.gov/dashboard/DTXSID40926674 | |
Description | DSSTox provides a high quality public chemistry resource for supporting improved predictive toxicology. | |
Synthesis routes and methods
Procedure details
試験管内研究製品の免責事項と情報
BenchChemで提示されるすべての記事および製品情報は、情報提供を目的としています。BenchChemで購入可能な製品は、生体外研究のために特別に設計されています。生体外研究は、ラテン語の "in glass" に由来し、生物体の外で行われる実験を指します。これらの製品は医薬品または薬として分類されておらず、FDAから任何の医療状態、病気、または疾患の予防、治療、または治癒のために承認されていません。これらの製品を人間または動物に体内に導入する形態は、法律により厳格に禁止されています。これらのガイドラインに従うことは、研究と実験において法的および倫理的な基準の遵守を確実にするために重要です。