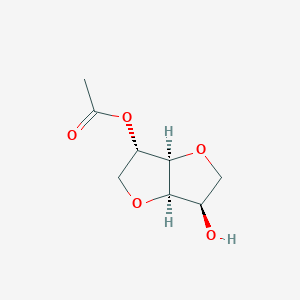
イソソルビド-2-アセテート
概要
説明
[(3R,3aR,6S,6aR)-3-hydroxy-2,3,3a,5,6,6a-hexahydrofuro[3,2-b]furan-6-yl] acetate is a derivative of 1,4:3,6-Dianhydro-D-glucitol, commonly known as isosorbide. This compound is of significant interest due to its potential applications in various fields, including pharmaceuticals, polymers, and as a building block for more complex chemical entities. It is characterized by its unique bicyclic structure, which imparts distinct chemical and physical properties.
科学的研究の応用
[(3R,3aR,6S,6aR)-3-hydroxy-2,3,3a,5,6,6a-hexahydrofuro[3,2-b]furan-6-yl] acetate has several scientific research applications:
作用機序
Target of Action
Isosorbide-2-acetate, also known as 1,4:3,6-Dianhydro-D-glucitol 2-acetate, is a derivative of isosorbide, which is an organic nitrate with vasodilating properties . The primary targets of isosorbide and its derivatives are the smooth muscles of both arteries and veins, predominantly veins . The compound’s action on these targets helps reduce cardiac preload .
Mode of Action
Isosorbide-2-acetate, like other organic nitrates, acts as a prodrug for nitric oxide (NO), which is a potent vasodilator gas . The compound is metabolized to release NO, which then interacts with its targets to mediate the therapeutic action of isosorbide-2-acetate . This interaction results in the relaxation of the vascular smooth muscle and consequent dilation of peripheral arteries and veins .
Biochemical Pathways
The biochemical pathways affected by isosorbide-2-acetate are primarily related to vasodilation and the regulation of blood pressure . The release of NO from the compound activates guanylate cyclase, leading to an increase in cyclic guanosine monophosphate (cGMP) in smooth muscle cells . This increase in cGMP then leads to the relaxation of smooth muscle cells, resulting in vasodilation .
Result of Action
The primary molecular and cellular effects of isosorbide-2-acetate’s action are the relaxation of vascular smooth muscle and the dilation of peripheral arteries and veins . These effects lead to a decrease in venous return to the heart, thereby reducing left ventricular end-diastolic pressure and pulmonary capillary wedge pressure . This can help in the management of conditions such as angina pectoris and congestive heart failure .
Action Environment
Environmental factors can influence the action, efficacy, and stability of isosorbide-2-acetate. For instance, factors such as pH and temperature could potentially affect the stability of the compound and its ability to release NO . Additionally, the presence of other medications or substances could potentially impact the compound’s absorption and metabolism, thereby affecting its efficacy . .
生化学分析
Biochemical Properties
Isosorbide-2-acetate plays a role in biochemical reactions, particularly as an intermediate in the synthesis of isosorbide-5-mononitrate . It interacts with enzymes such as lipase from Pseudomonas fluorescens in its synthesis . The nature of these interactions involves the enzymatic hydrolysis or alcoholysis of isosorbide-2,5-diacetate .
Cellular Effects
The cellular effects of Isosorbide-2-acetate are not well-studied. Its derivative, isosorbide, has been found to influence cell function. For instance, isosorbide reduces intraocular pressure through its effects on ocular blood vessels . While in the blood, isosorbide promotes redistribution of water toward the circulation, promoting the excretion of urine .
Molecular Mechanism
It is known that the synthesis of Isosorbide-2-acetate involves dissolving isosorbide-2,5-diacetate in acetone and n-heptanol, followed by the addition of lipase from Pseudomonas fluorescens . The mixture is stirred at room temperature for 2 days, after which the enzyme is removed by filtration .
Temporal Effects in Laboratory Settings
It is known that the synthesis of Isosorbide-2-acetate through enzymatic hydrolysis or alcoholysis yields a colorless crystalline substance with a melting point of 75°-76°C .
Metabolic Pathways
Isosorbide-2-acetate is involved in metabolic pathways related to the synthesis of isosorbide-5-mononitrate . This process involves the interaction of Isosorbide-2-acetate with enzymes such as lipase from Pseudomonas fluorescens .
Transport and Distribution
It is known that the compound can be synthesized within cells through enzymatic processes .
Subcellular Localization
Given its role as an intermediate in the synthesis of isosorbide-5-mononitrate, it is likely that it is localized in areas of the cell where this synthesis occurs .
準備方法
The synthesis of [(3R,3aR,6S,6aR)-3-hydroxy-2,3,3a,5,6,6a-hexahydrofuro[3,2-b]furan-6-yl] acetate typically involves the acetylation of 1,4:3,6-Dianhydro-D-glucitol. The process generally includes the following steps:
Acetylation Reaction: 1,4:3,6-Dianhydro-D-glucitol is reacted with acetic anhydride in the presence of an acid catalyst.
Industrial production methods may involve similar steps but are optimized for large-scale synthesis, including the use of continuous flow reactors and automated purification systems to enhance yield and efficiency.
化学反応の分析
[(3R,3aR,6S,6aR)-3-hydroxy-2,3,3a,5,6,6a-hexahydrofuro[3,2-b]furan-6-yl] acetate undergoes various chemical reactions, including:
The major products formed from these reactions depend on the specific reagents and conditions used. For example, oxidation typically yields carboxylic acids, while reduction produces alcohols.
類似化合物との比較
[(3R,3aR,6S,6aR)-3-hydroxy-2,3,3a,5,6,6a-hexahydrofuro[3,2-b]furan-6-yl] acetate can be compared with other similar compounds such as:
1,43,6-Dianhydro-D-glucitol 2-nitrate: This compound is used as a coronary vasodilator and has different pharmacological properties compared to the acetate derivative.
1,43,6-Dianhydro-D-glucitol 2,5-dinitrate: Known for its explosive properties, this compound is structurally similar but has distinct chemical reactivity and applications.
Dimethyl isosorbide: Used as a solvent and coalescent in various industrial applications, it differs in its functional groups and resultant properties.
The uniqueness of [(3R,3aR,6S,6aR)-3-hydroxy-2,3,3a,5,6,6a-hexahydrofuro[3,2-b]furan-6-yl] acetate lies in its specific acetyl group, which imparts unique reactivity and applications, particularly in the synthesis of biodegradable polymers and pharmaceuticals.
特性
IUPAC Name |
(3-hydroxy-2,3,3a,5,6,6a-hexahydrofuro[3,2-b]furan-6-yl) acetate | |
---|---|---|
Details | Computed by LexiChem 2.6.6 (PubChem release 2019.06.18) | |
Source | PubChem | |
URL | https://pubchem.ncbi.nlm.nih.gov | |
Description | Data deposited in or computed by PubChem | |
InChI |
InChI=1S/C8H12O5/c1-4(9)13-6-3-12-7-5(10)2-11-8(6)7/h5-8,10H,2-3H2,1H3 | |
Details | Computed by InChI 1.0.5 (PubChem release 2019.06.18) | |
Source | PubChem | |
URL | https://pubchem.ncbi.nlm.nih.gov | |
Description | Data deposited in or computed by PubChem | |
InChI Key |
ASUQMWUAFHPXOG-UHFFFAOYSA-N | |
Details | Computed by InChI 1.0.5 (PubChem release 2019.06.18) | |
Source | PubChem | |
URL | https://pubchem.ncbi.nlm.nih.gov | |
Description | Data deposited in or computed by PubChem | |
Canonical SMILES |
CC(=O)OC1COC2C1OCC2O | |
Details | Computed by OEChem 2.1.5 (PubChem release 2019.06.18) | |
Source | PubChem | |
URL | https://pubchem.ncbi.nlm.nih.gov | |
Description | Data deposited in or computed by PubChem | |
Molecular Formula |
C8H12O5 | |
Details | Computed by PubChem 2.1 (PubChem release 2019.06.18) | |
Source | PubChem | |
URL | https://pubchem.ncbi.nlm.nih.gov | |
Description | Data deposited in or computed by PubChem | |
DSSTOX Substance ID |
DTXSID80926734 | |
Record name | 2-O-Acetyl-1,4:3,6-dianhydrohexitol | |
Source | EPA DSSTox | |
URL | https://comptox.epa.gov/dashboard/DTXSID80926734 | |
Description | DSSTox provides a high quality public chemistry resource for supporting improved predictive toxicology. | |
Molecular Weight |
188.18 g/mol | |
Details | Computed by PubChem 2.1 (PubChem release 2021.05.07) | |
Source | PubChem | |
URL | https://pubchem.ncbi.nlm.nih.gov | |
Description | Data deposited in or computed by PubChem | |
CAS No. |
13042-39-2 | |
Record name | 1,4:3,6-Dianhydro-D-glucitol 2-acetate | |
Source | ChemIDplus | |
URL | https://pubchem.ncbi.nlm.nih.gov/substance/?source=chemidplus&sourceid=0013042392 | |
Description | ChemIDplus is a free, web search system that provides access to the structure and nomenclature authority files used for the identification of chemical substances cited in National Library of Medicine (NLM) databases, including the TOXNET system. | |
Record name | 2-O-Acetyl-1,4:3,6-dianhydrohexitol | |
Source | EPA DSSTox | |
URL | https://comptox.epa.gov/dashboard/DTXSID80926734 | |
Description | DSSTox provides a high quality public chemistry resource for supporting improved predictive toxicology. | |
Retrosynthesis Analysis
AI-Powered Synthesis Planning: Our tool employs the Template_relevance Pistachio, Template_relevance Bkms_metabolic, Template_relevance Pistachio_ringbreaker, Template_relevance Reaxys, Template_relevance Reaxys_biocatalysis model, leveraging a vast database of chemical reactions to predict feasible synthetic routes.
One-Step Synthesis Focus: Specifically designed for one-step synthesis, it provides concise and direct routes for your target compounds, streamlining the synthesis process.
Accurate Predictions: Utilizing the extensive PISTACHIO, BKMS_METABOLIC, PISTACHIO_RINGBREAKER, REAXYS, REAXYS_BIOCATALYSIS database, our tool offers high-accuracy predictions, reflecting the latest in chemical research and data.
Strategy Settings
Precursor scoring | Relevance Heuristic |
---|---|
Min. plausibility | 0.01 |
Model | Template_relevance |
Template Set | Pistachio/Bkms_metabolic/Pistachio_ringbreaker/Reaxys/Reaxys_biocatalysis |
Top-N result to add to graph | 6 |
Feasible Synthetic Routes
Q1: What is the significance of the regioselectivity achieved in the enzymatic synthesis of Isosorbide-2-acetate?
A1: The enzymatic synthesis of Isosorbide-2-acetate utilizes immobilized lipase, exhibiting remarkable regioselectivity favoring the 2-acetate isomer over the 5-acetate isomer [, ]. This selectivity is crucial because it simplifies the subsequent synthesis of Isosorbide-5-mononitrate, a valuable pharmaceutical compound. By achieving high regioselectivity (>99.5% d.e.), the need for complex and costly separation techniques is minimized, enhancing the efficiency and cost-effectiveness of the overall process [].
Q2: What are the advantages of using an enzymatic approach for the synthesis of Isosorbide-2-acetate compared to traditional chemical methods?
A2: The enzymatic synthesis of Isosorbide-2-acetate, utilizing immobilized lipase like Novozym 435 or lipase PS IM, offers several advantages over traditional chemical methods [, ]. Firstly, the enzymatic process proceeds under milder reaction conditions, reducing the formation of unwanted byproducts and simplifying purification []. Secondly, the high regioselectivity of the enzymes minimizes the production of the undesired Isosorbide-5-acetate isomer, streamlining downstream processing [, ]. Lastly, the use of immobilized enzymes allows for their recovery and reuse, contributing to a more sustainable and cost-effective process [].
Q3: Can you elaborate on the scalability of the enzymatic synthesis of Isosorbide-2-acetate and its implications for industrial applications?
A3: The research demonstrates the successful scaling up of the enzymatic synthesis of Isosorbide-2-acetate to a kilogram scale []. This scalability is a significant finding, indicating the potential of this enzymatic method for industrial production. The process maintains high yields (∼92%) and excellent regioselectivity (>99.5% d.e.) even at larger scales, highlighting its practicality for manufacturing Isosorbide-2-acetate as a key intermediate for pharmaceuticals like Isosorbide-5-mononitrate []. This scalability opens avenues for more efficient and sustainable production of this important pharmaceutical compound.
試験管内研究製品の免責事項と情報
BenchChemで提示されるすべての記事および製品情報は、情報提供を目的としています。BenchChemで購入可能な製品は、生体外研究のために特別に設計されています。生体外研究は、ラテン語の "in glass" に由来し、生物体の外で行われる実験を指します。これらの製品は医薬品または薬として分類されておらず、FDAから任何の医療状態、病気、または疾患の予防、治療、または治癒のために承認されていません。これらの製品を人間または動物に体内に導入する形態は、法律により厳格に禁止されています。これらのガイドラインに従うことは、研究と実験において法的および倫理的な基準の遵守を確実にするために重要です。