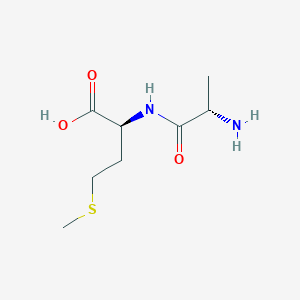
L-Alanyl-L-methionine
概要
説明
L-Alanyl-L-methionine is a dipeptide composed of the amino acids L-alanine and L-methionine. This compound is of interest due to its potential applications in various fields, including biochemistry, medicine, and nutrition. The combination of these two amino acids results in a molecule that exhibits unique properties, making it valuable for scientific research and industrial applications.
作用機序
Target of Action
L-Alanyl-L-methionine (Ala-Met) is a dipeptide composed of the amino acids alanine and methionine. The primary targets of Ala-Met are the enzymes involved in the metabolic pathways of these amino acids. Methionine, for instance, plays a crucial role in protein synthesis and is a precursor to S-adenosyl-methionine (SAMe), a universal methyl donor . Alanine, on the other hand, is rapidly metabolized via alanine aminotransferase to pyruvate, contributing to antioxidant defense .
Mode of Action
The mode of action of Ala-Met involves its interaction with the metabolic pathways of its constituent amino acids. Methionine is metabolized in the liver, leading to decreased levels of hepatic glutathione and increased oxidative stress . Alanine works in parallel with glutamine, sparing glutamine metabolism for high-demand tissues under inflammatory and highly catabolic situations .
Biochemical Pathways
Ala-Met affects several biochemical pathways. Methionine metabolism involves the formation of SAMe, L-homocysteine, L-cysteine, taurine, and sulfate . Alanine metabolism, on the other hand, contributes to the production of pyruvate and glutamate . These pathways have downstream effects on various cellular functions, including protein synthesis, antioxidant defense, and immune response.
Result of Action
The molecular and cellular effects of Ala-Met’s action are primarily related to its role in amino acid metabolism. By providing methionine and alanine, Ala-Met can support protein synthesis, antioxidant defense, and immune function . In addition, methionine metabolism can influence cellular methylation processes, while alanine metabolism can affect energy production and redox balance .
Action Environment
The action, efficacy, and stability of Ala-Met can be influenced by various environmental factors. For instance, the availability of other nutrients, the presence of specific enzymes, and the physiological state of the organism can all affect how Ala-Met is metabolized and utilized. Furthermore, the production of Ala-Met via microbial fermentation can be influenced by factors such as nutrient availability, pH, temperature, and the genetic characteristics of the microbial strain .
準備方法
Synthetic Routes and Reaction Conditions: L-Alanyl-L-methionine can be synthesized through a peptide coupling reaction between L-alanine and L-methionine. This process typically involves the use of coupling reagents such as dicyclohexylcarbodiimide (DCC) or N,N’-diisopropylcarbodiimide (DIC) in the presence of a base like N-methylmorpholine (NMM) or triethylamine (TEA). The reaction is carried out in an organic solvent such as dimethylformamide (DMF) or dichloromethane (DCM) under controlled temperature conditions.
Industrial Production Methods: Industrial production of this compound may involve enzymatic synthesis using amino acid ligases or proteases. These enzymes facilitate the formation of the peptide bond between L-alanine and L-methionine under mild conditions, making the process more environmentally friendly and efficient compared to traditional chemical synthesis.
化学反応の分析
Types of Reactions: L-Alanyl-L-methionine can undergo various chemical reactions, including:
Oxidation: The methionine residue can be oxidized to methionine sulfoxide or methionine sulfone using oxidizing agents such as hydrogen peroxide or performic acid.
Reduction: The compound can be reduced using reducing agents like sodium borohydride or lithium aluminum hydride.
Substitution: The amino and carboxyl groups can participate in substitution reactions, forming derivatives with different functional groups.
Common Reagents and Conditions:
Oxidation: Hydrogen peroxide, performic acid.
Reduction: Sodium borohydride, lithium aluminum hydride.
Substitution: Various acylating or alkylating agents.
Major Products Formed:
Oxidation: Methionine sulfoxide, methionine sulfone.
Reduction: Reduced forms of the dipeptide.
Substitution: Derivatives with modified amino or carboxyl groups.
科学的研究の応用
L-Alanyl-L-methionine has several applications in scientific research:
Biochemistry: Used as a model compound to study peptide bond formation and stability.
Medicine: Investigated for its potential therapeutic effects, including antioxidant properties and its role in protein synthesis.
Nutrition: Studied for its nutritional benefits, particularly in enhancing the bioavailability of methionine.
Industry: Utilized in the production of specialized nutritional supplements and pharmaceuticals.
類似化合物との比較
L-Alanyl-L-methionine can be compared with other dipeptides such as L-alanyl-L-glutamine and L-alanyl-L-tyrosine. While all these compounds share the common feature of containing L-alanine, their unique properties are derived from the second amino acid in the dipeptide:
L-Alanyl-L-glutamine: Known for its stability and use in clinical nutrition.
L-Alanyl-L-tyrosine: Studied for its potential cognitive benefits.
This compound stands out due to the presence of methionine, which imparts antioxidant properties and plays a crucial role in methylation reactions.
特性
IUPAC Name |
(2S)-2-[[(2S)-2-aminopropanoyl]amino]-4-methylsulfanylbutanoic acid | |
---|---|---|
Source | PubChem | |
URL | https://pubchem.ncbi.nlm.nih.gov | |
Description | Data deposited in or computed by PubChem | |
InChI |
InChI=1S/C8H16N2O3S/c1-5(9)7(11)10-6(8(12)13)3-4-14-2/h5-6H,3-4,9H2,1-2H3,(H,10,11)(H,12,13)/t5-,6-/m0/s1 | |
Source | PubChem | |
URL | https://pubchem.ncbi.nlm.nih.gov | |
Description | Data deposited in or computed by PubChem | |
InChI Key |
FSHURBQASBLAPO-WDSKDSINSA-N | |
Source | PubChem | |
URL | https://pubchem.ncbi.nlm.nih.gov | |
Description | Data deposited in or computed by PubChem | |
Canonical SMILES |
CC(C(=O)NC(CCSC)C(=O)O)N | |
Source | PubChem | |
URL | https://pubchem.ncbi.nlm.nih.gov | |
Description | Data deposited in or computed by PubChem | |
Isomeric SMILES |
C[C@@H](C(=O)N[C@@H](CCSC)C(=O)O)N | |
Source | PubChem | |
URL | https://pubchem.ncbi.nlm.nih.gov | |
Description | Data deposited in or computed by PubChem | |
Molecular Formula |
C8H16N2O3S | |
Source | PubChem | |
URL | https://pubchem.ncbi.nlm.nih.gov | |
Description | Data deposited in or computed by PubChem | |
DSSTOX Substance ID |
DTXSID50932428 | |
Record name | N-(2-Amino-1-hydroxypropylidene)methionine | |
Source | EPA DSSTox | |
URL | https://comptox.epa.gov/dashboard/DTXSID50932428 | |
Description | DSSTox provides a high quality public chemistry resource for supporting improved predictive toxicology. | |
Molecular Weight |
220.29 g/mol | |
Source | PubChem | |
URL | https://pubchem.ncbi.nlm.nih.gov | |
Description | Data deposited in or computed by PubChem | |
Physical Description |
Solid | |
Record name | Alanylmethionine | |
Source | Human Metabolome Database (HMDB) | |
URL | http://www.hmdb.ca/metabolites/HMDB0028693 | |
Description | The Human Metabolome Database (HMDB) is a freely available electronic database containing detailed information about small molecule metabolites found in the human body. | |
Explanation | HMDB is offered to the public as a freely available resource. Use and re-distribution of the data, in whole or in part, for commercial purposes requires explicit permission of the authors and explicit acknowledgment of the source material (HMDB) and the original publication (see the HMDB citing page). We ask that users who download significant portions of the database cite the HMDB paper in any resulting publications. | |
CAS No. |
14486-05-6 | |
Record name | L-Alanyl-L-methionine | |
Source | CAS Common Chemistry | |
URL | https://commonchemistry.cas.org/detail?cas_rn=14486-05-6 | |
Description | CAS Common Chemistry is an open community resource for accessing chemical information. Nearly 500,000 chemical substances from CAS REGISTRY cover areas of community interest, including common and frequently regulated chemicals, and those relevant to high school and undergraduate chemistry classes. This chemical information, curated by our expert scientists, is provided in alignment with our mission as a division of the American Chemical Society. | |
Explanation | The data from CAS Common Chemistry is provided under a CC-BY-NC 4.0 license, unless otherwise stated. | |
Record name | L-Alanyl-L-methionine | |
Source | ChemIDplus | |
URL | https://pubchem.ncbi.nlm.nih.gov/substance/?source=chemidplus&sourceid=0014486056 | |
Description | ChemIDplus is a free, web search system that provides access to the structure and nomenclature authority files used for the identification of chemical substances cited in National Library of Medicine (NLM) databases, including the TOXNET system. | |
Record name | N-(2-Amino-1-hydroxypropylidene)methionine | |
Source | EPA DSSTox | |
URL | https://comptox.epa.gov/dashboard/DTXSID50932428 | |
Description | DSSTox provides a high quality public chemistry resource for supporting improved predictive toxicology. | |
Record name | L-alanyl-L-methionine | |
Source | European Chemicals Agency (ECHA) | |
URL | https://echa.europa.eu/substance-information/-/substanceinfo/100.034.974 | |
Description | The European Chemicals Agency (ECHA) is an agency of the European Union which is the driving force among regulatory authorities in implementing the EU's groundbreaking chemicals legislation for the benefit of human health and the environment as well as for innovation and competitiveness. | |
Explanation | Use of the information, documents and data from the ECHA website is subject to the terms and conditions of this Legal Notice, and subject to other binding limitations provided for under applicable law, the information, documents and data made available on the ECHA website may be reproduced, distributed and/or used, totally or in part, for non-commercial purposes provided that ECHA is acknowledged as the source: "Source: European Chemicals Agency, http://echa.europa.eu/". Such acknowledgement must be included in each copy of the material. ECHA permits and encourages organisations and individuals to create links to the ECHA website under the following cumulative conditions: Links can only be made to webpages that provide a link to the Legal Notice page. | |
Record name | Alanylmethionine | |
Source | Human Metabolome Database (HMDB) | |
URL | http://www.hmdb.ca/metabolites/HMDB0028693 | |
Description | The Human Metabolome Database (HMDB) is a freely available electronic database containing detailed information about small molecule metabolites found in the human body. | |
Explanation | HMDB is offered to the public as a freely available resource. Use and re-distribution of the data, in whole or in part, for commercial purposes requires explicit permission of the authors and explicit acknowledgment of the source material (HMDB) and the original publication (see the HMDB citing page). We ask that users who download significant portions of the database cite the HMDB paper in any resulting publications. | |
Retrosynthesis Analysis
AI-Powered Synthesis Planning: Our tool employs the Template_relevance Pistachio, Template_relevance Bkms_metabolic, Template_relevance Pistachio_ringbreaker, Template_relevance Reaxys, Template_relevance Reaxys_biocatalysis model, leveraging a vast database of chemical reactions to predict feasible synthetic routes.
One-Step Synthesis Focus: Specifically designed for one-step synthesis, it provides concise and direct routes for your target compounds, streamlining the synthesis process.
Accurate Predictions: Utilizing the extensive PISTACHIO, BKMS_METABOLIC, PISTACHIO_RINGBREAKER, REAXYS, REAXYS_BIOCATALYSIS database, our tool offers high-accuracy predictions, reflecting the latest in chemical research and data.
Strategy Settings
Precursor scoring | Relevance Heuristic |
---|---|
Min. plausibility | 0.01 |
Model | Template_relevance |
Template Set | Pistachio/Bkms_metabolic/Pistachio_ringbreaker/Reaxys/Reaxys_biocatalysis |
Top-N result to add to graph | 6 |
Feasible Synthetic Routes
Q1: What is the molecular formula and weight of Ala-Met?
A1: The molecular formula of Ala-Met is C7H14N2O3S, and its molecular weight is 206.27 g/mol.
Q2: Is there any spectroscopic data available for Ala-Met?
A2: While the provided research doesn't delve into detailed spectroscopic characterization, techniques like nuclear magnetic resonance (NMR) spectroscopy can be employed to analyze its structure and properties. For instance, in a study on F pilin, 1H-nuclear magnetic resonance spectroscopy was used to determine the N-terminal alanine residue blocking by an acetyl group. []
Q3: What is the significance of the peptide bond in Ala-Met?
A3: The peptide bond linking alanine and methionine is crucial for the dipeptide's stability and biological activity. Studies have shown that specific reagents and conditions during peptide synthesis can lead to racemization, impacting its properties. []
Q4: Does Ala-Met play a role in the antigenic determinants of blood groups?
A4: Research suggests that the octapeptides containing Ala-Met at the N-terminus of glycophorin A contribute to the antigenic determinants of the M and N blood group systems. Specifically, the amino-terminal serine of the octapeptide in M-glycophorin A is a primary determinant for the M antigen. []
Q5: Has Ala-Met been identified in naturally occurring peptides with biological activity?
A5: Yes, Ala-Met is found within various biologically active peptides. For example, it's present in the sequence of somatostatin-28, a naturally occurring peptide found in ovine hypothalamic extracts, known for its somatotropin (growth hormone) and prolactin release-inhibiting activities. [, ]
Q6: How does the sequence context of Ala-Met affect its biological properties?
A6: The specific amino acid sequence surrounding Ala-Met significantly influences its biological activity. For instance, in studies on triticale carboxypeptidase III, N-CBZ-Ala-Met was hydrolyzed at a high rate, suggesting the enzyme's preference for aromatic or large aliphatic side chains adjacent to Ala-Met. []
Q7: Are there any known interactions between Ala-Met and metal ions?
A7: Research indicates that the presence of Ala-Met within specific peptide sequences can influence metal binding. A study focusing on the Al transporter Nrat1 highlighted the importance of the Ala-Met-Val-Met motif in metal binding and transport. Substituting this motif with one from a Mn transporter led to changes in Al transport activity, emphasizing the role of the motif in metal selectivity. []
Q8: What is the role of Ala-Met in enzyme-substrate interactions?
A8: Studies on carboxypeptidase III revealed that the presence of Ala-Met within a peptide substrate influences its hydrolysis rate. This suggests that the specific amino acid context around Ala-Met can either enhance or hinder enzyme recognition and activity. []
Q9: How stable is Ala-Met under various conditions?
A9: While the provided research doesn't directly address Ala-Met's stability, general peptide stability is influenced by factors like pH, temperature, and enzymatic degradation.
Q10: Are there specific formulation strategies to improve Ala-Met stability?
A10: Although not explicitly discussed for Ala-Met, common peptide formulation strategies, such as lyophilization, the use of stabilizing excipients, and encapsulation techniques, can potentially enhance its stability. For example, in the synthesis of prosomatostatin, a putative somatostatin precursor, the peptide was purified using techniques like gel filtration and ion-exchange chromatography to obtain a stable and pure product for biological testing. []
Q11: What are the potential applications of Ala-Met in drug development?
A11: Understanding the role of Ala-Met within bioactive peptides can guide the development of novel therapeutics. For instance, research on synthetic peptides like LT-10, derived from Lethal Toxin Neutralizing Factor (LTNF), shows promise in treating intoxication caused by various toxins. [, ] Further research is necessary to explore its full therapeutic potential in areas like anti-venom and anti-toxin therapy.
試験管内研究製品の免責事項と情報
BenchChemで提示されるすべての記事および製品情報は、情報提供を目的としています。BenchChemで購入可能な製品は、生体外研究のために特別に設計されています。生体外研究は、ラテン語の "in glass" に由来し、生物体の外で行われる実験を指します。これらの製品は医薬品または薬として分類されておらず、FDAから任何の医療状態、病気、または疾患の予防、治療、または治癒のために承認されていません。これらの製品を人間または動物に体内に導入する形態は、法律により厳格に禁止されています。これらのガイドラインに従うことは、研究と実験において法的および倫理的な基準の遵守を確実にするために重要です。