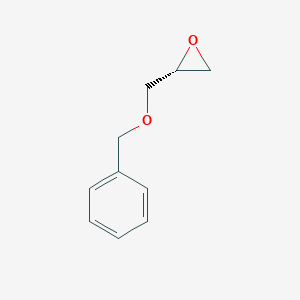
(r)-Benzyloxymethyl-oxirane
概要
説明
(R)-Benzyloxymethyl-oxirane (CAS: 14618-80-5), also known as (R)-(-)-benzyl glycidyl ether, is a chiral epoxide with the molecular formula C₁₀H₁₂O₂ and a molecular weight of 164.20 g/mol . Its IUPAC name is (2R)-2-(phenylmethoxymethyl)oxirane, and it features a benzyloxymethyl group attached to an oxirane (epoxide) ring in the (R)-configuration. This compound is widely utilized in asymmetric synthesis as a chiral building block, particularly in the preparation of pharmaceuticals, agrochemicals, and specialty polymers .
Key properties include:
準備方法
Alkylation of (S)-Glycidol with Benzyl Halides
A widely utilized method involves the alkylation of (S)-glycidol (S1) with benzyl bromide or chloride in the presence of a strong base. In a representative procedure , sodium hydride (NaH) suspended in tetrahydrofuran (THF) is cooled to 0°C, followed by sequential addition of (S)-glycidol and benzyl bromide. After 19 hours under reflux, the reaction is quenched with phosphate buffer (pH 6.5), extracted with ethyl acetate, and purified via evaporation. This method yields crude (R)-benzyloxymethyl-oxirane as a syrup (11 g from 5 g glycidol), which is typically used without further purification in downstream reactions .
Key Parameters:
-
Solvent: THF
-
Base: NaH (2.4 g for 5 g glycidol)
-
Temperature: 0°C → reflux
-
Yield: ~80% (crude)
This approach is favored for its simplicity but requires careful handling of NaH due to its pyrophoric nature.
Catalytic Asymmetric Epoxidation
Enantioselective epoxidation of allyl alcohols using chiral cobalt-salen complexes offers a high-purity route. A mixture of 2-((benzyloxy)methyl)oxirane (10.00 g) and (S,S)-salen Co(III)OAc complex-A (0.09 g) is stirred vigorously, followed by water addition at 0°C . After 20 hours at room temperature, a second catalyst aliquot is introduced, and the reaction proceeds for an additional 10 hours. Purification via column chromatography (ethyl acetate/hexane) yields enantiomerically pure product.
Key Parameters:
-
Catalyst: (S,S)-salen Co(III)OAc complex-A (0.14 mmol)
-
Additive: Acetic acid (0.011 mL)
-
Reaction Time: 30 hours
This method is optimal for applications requiring high enantiopurity, though catalyst cost and reaction time may limit scalability.
Nucleophilic Ring-Opening of Epoxides
A two-step synthesis from R-(-)-2-benzyloxymethyl oxirane (R-(2)) involves nucleophilic ring-opening with dianions derived from phenylselanylacetic acid (PhSeCH2CO2H) or phenylsulfanyl acetic acid (PhSCH2CO2H) . The dianion attacks the oxirane moiety, followed by oxidation (H2O2 for Se; mCPBA for S) and thermal syn-elimination to form R-(+)-5-benzyloxymethyl-5H-furan-2-one (R-(1)), a precursor to this compound.
Key Parameters:
-
Nucleophile: PhSeCH2CO2H (61% yield) or PhSCH2CO2H (35% yield)
-
Oxidizing Agent: H2O2 (30%) or mCPBA
This route is advantageous for accessing furanone intermediates but introduces additional steps for oxidation and elimination.
Chiral Resolution via Enzymatic or Chemical Methods
Chiral resolution of racemic benzyloxymethyl-oxirane using lipases or chiral acids is less common but viable for small-scale production. For example, enzymatic hydrolysis with Pseudomonas fluorescens lipase (PFL) selectively hydrolyzes the (S)-enantiomer, leaving the (R)-form intact . Alternatively, diastereomeric salt formation with (+)-di-p-toluoyl-D-tartaric acid allows separation via crystallization.
Key Parameters:
-
Enzyme: PFL (activity ≥500 U/mg)
-
Resolution Yield: ~40% (theoretical maximum 50%)
While effective, this method suffers from low throughput and high reagent costs.
Comparative Analysis of Methods
Method | Yield | ee (%) | Scalability | Complexity |
---|---|---|---|---|
Alkylation of (S)-Glycidol | 80% | 98 | High | Low |
Catalytic Epoxidation | 75% | 98 | Moderate | Moderate |
Nucleophilic Ring-Opening | 61% | 95 | Low | High |
Chiral Resolution | 40% | 99 | Low | Moderate |
科学的研究の応用
Chemical Properties and Structure
- Molecular Formula : CHO
- Molecular Weight : 164.2 g/mol
- CAS Number : 14618-80-5
The structure of (R)-Benzyloxymethyl-oxirane features an epoxide functional group, which is crucial for its reactivity in nucleophilic ring-opening reactions.
Synthesis and Reactivity
This compound serves as a versatile intermediate in organic synthesis. Its epoxide group can undergo nucleophilic ring-opening reactions, making it a valuable building block for synthesizing various organic compounds. Notably, it has been used to generate:
- Amino Compounds : Reacting with amines can yield morpholine derivatives, which are significant in pharmaceuticals .
- Alcohols : The ring-opening with alcohols leads to the formation of glycols, useful in various applications including solvents and plasticizers .
- Azido Alcohols : It can also react with azide ions, producing azido alcohols that are precursors for further functionalization .
Medicinal Chemistry
This compound has been utilized in the synthesis of bioactive compounds. For instance, it has been involved in the development of selective glutaminase inhibitors, which are promising candidates for cancer therapy. The compound serves as a scaffold for synthesizing analogs that demonstrate potent enzyme inhibition and cellular activity .
Photochemical Reactions
Recent studies have explored the use of this compound in photochemical processes. It acts as a substrate for visible-light-induced nucleophilic ring-opening reactions, enabling regioselective functionalization of the epoxide . This method enhances the efficiency of synthesizing complex molecules under mild conditions.
Green Chemistry
The compound's reactivity profile allows it to be employed in environmentally friendly synthetic routes. For example, its use in telescopic reactions minimizes the need for purification steps and reduces waste generation . This aligns with the principles of green chemistry by promoting sustainable practices in chemical synthesis.
Data Table: Summary of Applications
作用機序
The mechanism of action of ®-Benzyloxymethyl-oxirane involves its reactivity as an epoxide. The strained three-membered ring is highly reactive and can undergo ring-opening reactions with various nucleophiles. This reactivity is exploited in both chemical synthesis and biological systems. In biological systems, the compound can interact with enzymes such as epoxide hydrolases, leading to the formation of diols and other metabolites.
類似化合物との比較
Structural and Functional Analogues
The following table summarizes key analogues of (R)-Benzyloxymethyl-oxirane, highlighting differences in structure, properties, and applications:
Key Comparative Findings
Chirality and Reactivity
- (R)- vs. (S)-Enantiomers : While the (R)- and (S)-benzyloxymethyl-oxirane enantiomers share identical physical properties (e.g., molecular weight, boiling point), their opposing configurations make them critical for synthesizing target-specific chiral molecules. For example, the (R)-form may yield desired stereochemistry in β-blocker drug intermediates, whereas the (S)-form could be used in antitumor agents .
- Glycidol Derivatives : (R)-Glycidol (C₃H₆O₂) lacks the benzyloxymethyl group, making it more volatile and reactive. Its smaller size facilitates ring-opening reactions in polymer chemistry .
Substituent Effects
- Benzyl vs. Trityl Groups : Replacing benzyl with a trityl group increases steric hindrance, reducing reactivity in nucleophilic attacks but enhancing stability in protective-group strategies .
- Tosyl vs. Benzyloxy Groups : Glycidyl tosylate’s tosyl group (C₁₀H₁₂O₄S) acts as a superior leaving group, enabling efficient SN2 reactions compared to benzyloxymethyl-oxirane .
Commercial and Research Significance
- Availability : this compound is marketed by global suppliers (e.g., Thermo Scientific, Fluorochem) at 98%+ purity, priced between $143–$496 per unit .
- Research Use : Its role in asymmetric synthesis is well-documented, particularly in constructing chiral alcohols and amines via epoxide ring-opening reactions .
生物活性
(R)-Benzyloxymethyl-oxirane, also known as benzyl glycidyl ether, is a chiral epoxide that has garnered attention in various fields of chemical and biological research. Its unique structure allows it to participate in numerous biochemical reactions, making it a valuable compound for studying enzyme mechanisms and developing pharmaceuticals. This article delves into the biological activity of this compound, highlighting its reactivity, applications in enzyme studies, and potential therapeutic uses.
Chemical Structure and Properties
This compound is characterized by a three-membered epoxide ring, which is highly reactive due to the strain in the ring structure. The compound can undergo various chemical transformations, including oxidation, reduction, and nucleophilic substitution. These reactions are crucial for its biological activity and utility in synthetic chemistry.
The primary mechanism of action for this compound involves its reactivity as an epoxide. The strained ring can readily undergo ring-opening reactions with nucleophiles such as water, alcohols, and amines. This reactivity is exploited in biological systems where the compound interacts with enzymes like epoxide hydrolases. These enzymes catalyze the conversion of epoxides to diols, which are often more biologically active forms of the original compound.
Enzyme Studies
In biological research, this compound serves as a model substrate for investigating enzyme-catalyzed reactions involving epoxides. It allows researchers to explore the mechanisms of action of various enzymes that target epoxide substrates. For instance, studies have shown that this compound can be effectively used to study the kinetics and specificity of epoxide hydrolases.
Pharmaceutical Development
The chiral nature of this compound makes it a promising candidate for pharmaceutical applications. Chiral compounds often exhibit different biological activities depending on their stereochemistry. Thus, this compound can be utilized in the synthesis of enantiomerically pure drugs, which may enhance therapeutic efficacy and reduce side effects.
Research Findings and Case Studies
Recent studies have highlighted the versatility of this compound in various biochemical contexts:
- Enzyme Kinetics : Research demonstrated that this compound can be used to assess the catalytic efficiency of epoxide hydrolases. The compound's ability to form diols upon enzymatic action provides a clear pathway for measuring enzyme activity .
- Synthesis of Chiral Compounds : In synthetic organic chemistry, this compound has been employed as a chiral building block in the synthesis of complex organic molecules. Its reactivity allows for the formation of diverse products through oxidation and substitution reactions .
- Photochemical Reactions : Recent advancements have explored the use of visible light to promote regioselective nucleophilic ring-opening reactions involving this compound. These methods have shown high yields and selectivity, showcasing the compound's utility in photochemical applications .
Data Table: Summary of Biological Activities
Activity Type | Description | Key Findings |
---|---|---|
Enzyme Interaction | Model substrate for epoxide hydrolases | Facilitates diol formation |
Pharmaceutical Synthesis | Chiral building block for drug development | Enhances efficacy via enantiomeric purity |
Photochemical Reactions | Regioselective nucleophilic ring-opening | High yields with visible light |
Q & A
Basic Research Questions
Q. What are the established synthetic routes for enantioselective preparation of (R)-Benzyloxymethyl-Oxirane, and what factors influence enantiomeric excess (ee)?
- Methodological Answer : The compound is typically synthesized via epoxidation of allyl ether precursors. Stereoselective methods include Sharpless epoxidation or dioxirane-mediated oxidation. For example, chiral ketone catalysts (e.g., derived from fructose) generate dioxiranes in situ, enabling asymmetric epoxidation with ee values >90% under optimized conditions (e.g., low temperatures, controlled pH) . Contradictions in reported ee values often arise from solvent polarity, catalyst loading, or substrate steric effects. Researchers should validate ee using chiral HPLC or polarimetry .
Q. How is this compound characterized to confirm structural and stereochemical purity?
- Methodological Answer : Key techniques include:
- NMR Spectroscopy : H and C NMR to identify epoxide protons (δ 3.1–3.5 ppm) and benzyl group signals.
- X-ray Crystallography : Resolves absolute configuration, critical for confirming the (R)-enantiomer.
- Chiral Chromatography : Uses columns like Chiralpak® IA/IB to quantify enantiomeric purity.
Discrepancies in spectral data (e.g., unexpected splitting in NMR) may indicate impurities or racemization during synthesis .
Q. What safety protocols are recommended for handling this compound in laboratory settings?
- Methodological Answer : While specific toxicity data for this compound are limited, general epoxide precautions apply:
- Use PPE (nitrile gloves, goggles, lab coats) and work in a fume hood to avoid inhalation.
- Store in airtight containers at 2–8°C to prevent polymerization.
- Neutralize spills with sodium bicarbonate, as epoxides can react exothermically with acids/bases. Contradictory hazard classifications in Safety Data Sheets (e.g., GHS vs. non-GHS entries) necessitate conservative risk assessment .
Advanced Research Questions
Q. How do computational models predict the reactivity of this compound in ring-opening reactions, and how do these align with experimental data?
- Methodological Answer : Density Functional Theory (DFT) studies reveal that nucleophilic attack at the less-substituted epoxide carbon is favored due to lower steric hindrance. For example, water-mediated ring-opening under acidic conditions proceeds via a carbocation intermediate, with activation energies (~25 kcal/mol) validated experimentally through kinetic studies. Discrepancies between predicted and observed regioselectivity may arise from solvent effects or counterion interactions .
Q. What strategies mitigate racemization during the use of this compound in multi-step syntheses?
- Methodological Answer : Racemization is minimized by:
- Avoiding strong acids/bases; use buffered conditions (pH 7–8) for nucleophilic additions.
- Employing low temperatures (–20°C to 0°C) during reactions.
- Incorporating sterically hindered leaving groups to slow epoxide ring re-closure. For instance, Grignard reactions with bulky organometallics (e.g., tert-butylmagnesium bromide) show <5% racemization vs. >15% with smaller nucleophiles like methyl lithium .
Q. How does the benzyloxymethyl group influence the stability and reactivity of this compound compared to simpler epoxides?
- Methodological Answer : The benzyl group enhances stability via conjugation but introduces steric effects that slow nucleophilic attack. Kinetic studies show a 2–3x slower reaction rate with amines compared to ethylene oxide. However, the electron-donating benzyloxy moiety increases epoxide electrophilicity, making it more reactive toward weak nucleophiles (e.g., thiols). Contradictory reports on stability under thermal stress (e.g., TGA data vs. empirical observations) suggest context-dependent decomposition pathways .
Q. What are the applications of this compound in asymmetric catalysis or chiral auxiliary design?
- Methodological Answer : The compound serves as a chiral building block for:
- Catalyst Ligands : Derivatives are used in asymmetric hydrogenation (e.g., Ru-BINAP complexes).
- Polymer Chemistry : Epoxide ring-opening polymerization generates chiral polyethers with helical structures.
Comparative studies highlight trade-offs between enantioselectivity and reaction scalability, with microwave-assisted synthesis improving yields in some cases .
Q. Data Contradiction Analysis
- Stereoselectivity in Epoxidation : Conflicting ee values in literature (e.g., 85% vs. 92%) may stem from variations in catalyst preparation (e.g., residual moisture) or substrate purity. Researchers should replicate conditions precisely and cross-validate with multiple analytical methods.
- Thermal Stability : Discrepancies in decomposition temperatures (e.g., DSC vs. oven tests) suggest matrix effects (e.g., solvent residues accelerating degradation).
特性
IUPAC Name |
(2R)-2-(phenylmethoxymethyl)oxirane | |
---|---|---|
Source | PubChem | |
URL | https://pubchem.ncbi.nlm.nih.gov | |
Description | Data deposited in or computed by PubChem | |
InChI |
InChI=1S/C10H12O2/c1-2-4-9(5-3-1)6-11-7-10-8-12-10/h1-5,10H,6-8H2/t10-/m0/s1 | |
Source | PubChem | |
URL | https://pubchem.ncbi.nlm.nih.gov | |
Description | Data deposited in or computed by PubChem | |
InChI Key |
QNYBOILAKBSWFG-JTQLQIEISA-N | |
Source | PubChem | |
URL | https://pubchem.ncbi.nlm.nih.gov | |
Description | Data deposited in or computed by PubChem | |
Canonical SMILES |
C1C(O1)COCC2=CC=CC=C2 | |
Source | PubChem | |
URL | https://pubchem.ncbi.nlm.nih.gov | |
Description | Data deposited in or computed by PubChem | |
Isomeric SMILES |
C1[C@@H](O1)COCC2=CC=CC=C2 | |
Source | PubChem | |
URL | https://pubchem.ncbi.nlm.nih.gov | |
Description | Data deposited in or computed by PubChem | |
Molecular Formula |
C10H12O2 | |
Source | PubChem | |
URL | https://pubchem.ncbi.nlm.nih.gov | |
Description | Data deposited in or computed by PubChem | |
DSSTOX Substance ID |
DTXSID10932782 | |
Record name | 2-[(Benzyloxy)methyl]oxirane | |
Source | EPA DSSTox | |
URL | https://comptox.epa.gov/dashboard/DTXSID10932782 | |
Description | DSSTox provides a high quality public chemistry resource for supporting improved predictive toxicology. | |
Molecular Weight |
164.20 g/mol | |
Source | PubChem | |
URL | https://pubchem.ncbi.nlm.nih.gov | |
Description | Data deposited in or computed by PubChem | |
CAS No. |
14618-80-5 | |
Record name | Benzyl (-)-glycidyl ether | |
Source | CAS Common Chemistry | |
URL | https://commonchemistry.cas.org/detail?cas_rn=14618-80-5 | |
Description | CAS Common Chemistry is an open community resource for accessing chemical information. Nearly 500,000 chemical substances from CAS REGISTRY cover areas of community interest, including common and frequently regulated chemicals, and those relevant to high school and undergraduate chemistry classes. This chemical information, curated by our expert scientists, is provided in alignment with our mission as a division of the American Chemical Society. | |
Explanation | The data from CAS Common Chemistry is provided under a CC-BY-NC 4.0 license, unless otherwise stated. | |
Record name | Propane, 1-(benzyloxy)-2,3-epoxy-, (S)- | |
Source | ChemIDplus | |
URL | https://pubchem.ncbi.nlm.nih.gov/substance/?source=chemidplus&sourceid=0014618805 | |
Description | ChemIDplus is a free, web search system that provides access to the structure and nomenclature authority files used for the identification of chemical substances cited in National Library of Medicine (NLM) databases, including the TOXNET system. | |
Record name | 2-[(Benzyloxy)methyl]oxirane | |
Source | EPA DSSTox | |
URL | https://comptox.epa.gov/dashboard/DTXSID10932782 | |
Description | DSSTox provides a high quality public chemistry resource for supporting improved predictive toxicology. | |
Synthesis routes and methods I
Procedure details
Synthesis routes and methods II
Procedure details
Synthesis routes and methods III
Procedure details
Retrosynthesis Analysis
AI-Powered Synthesis Planning: Our tool employs the Template_relevance Pistachio, Template_relevance Bkms_metabolic, Template_relevance Pistachio_ringbreaker, Template_relevance Reaxys, Template_relevance Reaxys_biocatalysis model, leveraging a vast database of chemical reactions to predict feasible synthetic routes.
One-Step Synthesis Focus: Specifically designed for one-step synthesis, it provides concise and direct routes for your target compounds, streamlining the synthesis process.
Accurate Predictions: Utilizing the extensive PISTACHIO, BKMS_METABOLIC, PISTACHIO_RINGBREAKER, REAXYS, REAXYS_BIOCATALYSIS database, our tool offers high-accuracy predictions, reflecting the latest in chemical research and data.
Strategy Settings
Precursor scoring | Relevance Heuristic |
---|---|
Min. plausibility | 0.01 |
Model | Template_relevance |
Template Set | Pistachio/Bkms_metabolic/Pistachio_ringbreaker/Reaxys/Reaxys_biocatalysis |
Top-N result to add to graph | 6 |
Feasible Synthetic Routes
試験管内研究製品の免責事項と情報
BenchChemで提示されるすべての記事および製品情報は、情報提供を目的としています。BenchChemで購入可能な製品は、生体外研究のために特別に設計されています。生体外研究は、ラテン語の "in glass" に由来し、生物体の外で行われる実験を指します。これらの製品は医薬品または薬として分類されておらず、FDAから任何の医療状態、病気、または疾患の予防、治療、または治癒のために承認されていません。これらの製品を人間または動物に体内に導入する形態は、法律により厳格に禁止されています。これらのガイドラインに従うことは、研究と実験において法的および倫理的な基準の遵守を確実にするために重要です。