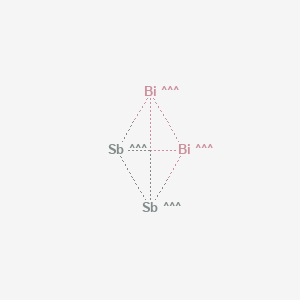
ビスマスアンチモニド (BiSb)
- 専門家チームからの見積もりを受け取るには、QUICK INQUIRYをクリックしてください。
- 品質商品を競争力のある価格で提供し、研究に集中できます。
説明
科学的研究の応用
Bismuth antimonide has a wide range of scientific research applications due to its unique properties:
Chemistry: It is used in the study of electronic structures and as a catalyst in certain chemical reactions.
作用機序
Target of Action
Bismuth antimonide (BiSb) is a binary alloy of bismuth and antimony in various ratios . It is a well-known thermoelectric material and a first-generation topological insulator at ambient conditions . The primary targets of BiSb are the electronic structures within the material, specifically the conduction and valence bands .
Mode of Action
BiSb interacts with its targets by altering the energy levels of the conduction and valence bands. When bismuth is doped with antimony, the conduction band decreases in energy and the valence band increases in energy . At an antimony concentration of 4%, the two bands intersect, forming a Dirac point . Further increases in the concentration of antimony result in a band inversion, in which the energy of the valence band becomes greater than that of the conduction band at specific momenta .
Biochemical Pathways
Two structural phase transitions are found at 8.5 and 20.3 GPa . The first anomaly is caused by the formation of the Weyl semimetallic state, which is attributed to a Bi–Sb bond between layers . The subsequent two are attributed to the trigonal to tetragonal and tetragonal to cubic phase transitions .
Pharmacokinetics
It’s important to note that bisb has low solubility , which could impact its bioavailability if it were to be used in a biological context.
Result of Action
The result of BiSb’s action is the transformation of the material into different phases under pressure . At higher concentrations of Sb, the band gap in the surface states vanishes, and the material thus conducts at its surface . This makes BiSb a topological insulator, materials that have conducting surface states but have an insulating interior .
準備方法
Synthetic Routes and Reaction Conditions
Bismuth antimonide can be synthesized by melting bismuth and antimony together under an inert gas or vacuum. The process involves heating the two elements until they form a homogeneous liquid, which is then cooled to form the alloy. Zone melting is often used to reduce impurities and achieve high-purity crystals .
Industrial Production Methods
In industrial settings, the production of bismuth antimonide typically involves large-scale melting and casting processes. The materials are melted in a controlled environment to prevent oxidation and contamination. The molten alloy is then cast into ingots or other desired shapes and allowed to cool slowly to ensure uniformity and minimize defects .
化学反応の分析
Types of Reactions
Bismuth antimonide undergoes various chemical reactions, including oxidation, reduction, and substitution. These reactions are influenced by the specific conditions and reagents used.
Common Reagents and Conditions
Oxidation: Bismuth antimonide can be oxidized using strong oxidizing agents such as nitric acid or oxygen at elevated temperatures.
Reduction: Reduction reactions typically involve hydrogen or other reducing agents to convert the compound back to its elemental forms.
Substitution: Substitution reactions can occur with halogens or other reactive species, leading to the formation of halide compounds.
Major Products Formed
The major products formed from these reactions include bismuth oxide, antimony oxide, and various halide compounds, depending on the specific reagents and conditions used .
類似化合物との比較
Similar Compounds
Bismuth oxide (Bi2O3): Known for its high refractive index and use in optical coatings.
Antimony trioxide (Sb2O3): Used as a flame retardant and in the production of glass and ceramics.
Bismuth telluride (Bi2Te3): Widely used in thermoelectric devices for cooling and power generation.
Uniqueness
Bismuth antimonide stands out due to its combination of bismuth and antimony, which imparts unique electronic properties not found in other bismuth or antimony compounds. Its ability to act as a topological insulator and its potential for use in advanced electronic applications make it a compound of significant interest .
生物活性
Bismuth antimonide (BiSb) is a compound that has garnered attention in the fields of materials science and medicinal chemistry due to its unique properties and biological activities. This article explores the biological activity of BiSb, focusing on its antimicrobial, anticancer, and antiparasitic properties, as well as its mechanisms of action. The information presented here is derived from a comprehensive review of recent studies and findings related to BiSb and its derivatives.
Bismuth antimonide is a binary compound formed from bismuth (Bi) and antimony (Sb). It exhibits semiconducting properties, which have made it a subject of interest in various applications, including thermoelectrics and optoelectronics. However, its biological activity is equally significant, particularly in the context of antimicrobial and anticancer research.
Property | Value |
---|---|
Molecular Formula | BiSb |
Melting Point | 540 °C |
Density | 9.78 g/cm³ |
Band Gap | ~0.2 eV |
Structure | Cubic |
Antimicrobial Activity
Bismuth compounds, including BiSb, have demonstrated significant antimicrobial properties, particularly against Helicobacter pylori, a bacterium associated with gastric ulcers and cancer. Studies show that BiSb can disrupt bacterial cell functions through various mechanisms:
- Inhibition of Enzymatic Activity : Bismuth can inactivate critical enzymes involved in bacterial respiration and metabolism, such as urease and alcohol dehydrogenase .
- Disruption of Membrane Integrity : Bismuth compounds induce the formation of membrane vesicles that facilitate the expulsion of toxic substances from bacterial cells .
- Multi-target Mechanisms : Recent research indicates that bismuth affects multiple pathways within bacteria, including oxidative stress response and pH-buffering capabilities .
Case Study: Efficacy Against H. pylori
A study conducted by Yao et al. utilized proteomic and metabolomic analyses to assess the effects of bismuth on six H. pylori strains. The findings revealed that bismuth inhibited growth by downregulating virulence factors such as CagA and VacA and disrupting flagella assembly essential for colonization .
Anticancer Activity
Bismuth compounds have also shown promise in cancer treatment. They exhibit cytotoxic effects against various cancer cell lines through mechanisms such as:
- Induction of Apoptosis : Bismuth has been reported to trigger programmed cell death in tumor cells by activating apoptotic pathways .
- Inhibition of Tumor Growth : Some studies suggest that bismuth compounds can inhibit tumor proliferation by interfering with cellular signaling pathways involved in growth regulation .
Antiparasitic Activity
Research indicates that bismuth compounds possess antiparasitic properties, particularly against protozoan infections such as leishmaniasis. The mechanisms include:
- Disruption of Parasite Metabolism : Bismuth interferes with metabolic pathways critical for parasite survival.
- Enhancement of Host Immune Response : Bismuth may modulate immune responses, enhancing the host's ability to combat parasitic infections .
Table 2: Summary of Biological Activities
Activity Type | Target Organisms | Mechanisms |
---|---|---|
Antimicrobial | H. pylori | Enzyme inhibition, membrane disruption |
Anticancer | Various cancer cell lines | Apoptosis induction, tumor growth inhibition |
Antiparasitic | Protozoan parasites | Metabolic disruption, immune modulation |
Structure-Activity Relationship (SAR)
The biological activity of bismuth compounds is influenced by their chemical structure. Research has highlighted the importance of ligand coordination in determining efficacy:
- Ligand Types : Different ligands can enhance or reduce the biological activity of bismuth complexes.
- Coordination Geometry : The spatial arrangement of ligands around the bismuth ion affects interaction with biological targets .
Case Study: Dithiocarbamate Complexes
Dithiocarbamate complexes of bismuth have shown significant antibacterial and anticancer activities. The structural diversity provided by dithiocarbamate ligands allows for tailored interactions with biological systems, enhancing therapeutic potential .
特性
CAS番号 |
12323-19-2 |
---|---|
分子式 |
BiSb |
分子量 |
330.740 g/mol |
IUPAC名 |
antimony;bismuth |
InChI |
InChI=1S/Bi.Sb |
InChIキー |
PEEDYJQEMCKDDX-UHFFFAOYSA-N |
SMILES |
[Sb].[Sb].[Bi].[Bi] |
正規SMILES |
[Sb].[Bi] |
物理的記述 |
Crystals; [MSDSonline] |
製品の起源 |
United States |
試験管内研究製品の免責事項と情報
BenchChemで提示されるすべての記事および製品情報は、情報提供を目的としています。BenchChemで購入可能な製品は、生体外研究のために特別に設計されています。生体外研究は、ラテン語の "in glass" に由来し、生物体の外で行われる実験を指します。これらの製品は医薬品または薬として分類されておらず、FDAから任何の医療状態、病気、または疾患の予防、治療、または治癒のために承認されていません。これらの製品を人間または動物に体内に導入する形態は、法律により厳格に禁止されています。これらのガイドラインに従うことは、研究と実験において法的および倫理的な基準の遵守を確実にするために重要です。