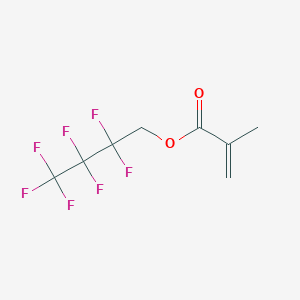
2,2,3,3,4,4,4-Heptafluorobutyl methacrylate
概要
説明
2,2,3,3,4,4,4-Heptafluorobutyl methacrylate: is a fluorinated monomer with the chemical formula C8H7F7O2 . It is known for its unique properties, including high thermal stability, chemical inertness, and low surface energy. These characteristics make it a valuable compound in various industrial and scientific applications .
準備方法
Synthetic Routes and Reaction Conditions: The synthesis of 2,2,3,3,4,4,4-Heptafluorobutyl methacrylate typically involves the esterification of methacrylic acid with 2,2,3,3,4,4,4-heptafluorobutanol. The reaction is usually catalyzed by an acid catalyst such as sulfuric acid or p-toluenesulfonic acid. The reaction is carried out under reflux conditions to ensure complete conversion of the reactants .
Industrial Production Methods: In industrial settings, the production of this compound follows similar synthetic routes but on a larger scale. The process involves the use of continuous reactors to maintain consistent reaction conditions and high yield. The product is then purified through distillation to remove any unreacted starting materials and by-products .
化学反応の分析
Polymerization Reactions
HFMA participates in both homopolymerization and copolymerization reactions, forming materials with enhanced thermal stability and chemical resistance.
Homopolymerization
Under free-radical initiation (e.g., azobisisobutyronitrile, AIBN), HFMA forms homopolymers with glass transition temperatures () between 65–75°C and decomposition temperatures exceeding 300°C .
Copolymerization
HFMA is frequently copolymerized with non-fluorinated monomers to balance fluorocarbon properties with processability:
-
Styrene-HFMA Copolymers : Exhibit values up to 105°C and water contact angles >110° , indicating high hydrophobicity .
-
Methyl Methacrylate (MMA)-HFMA Copolymers : Demonstrate improved thermal stability (decomposition onset at 280°C ) compared to pure PMMA .
Table 1: Thermal Properties of HFMA Copolymers
Monomer Combination | (°C) | Decomposition Onset (°C) | Water Contact Angle (°) |
---|---|---|---|
HFMA-Styrene | 105 | 310 | 112 |
HFMA-MMA | 89 | 280 | 98 |
HFMA-Butyl Acrylate | 72 | 265 | 105 |
Chemical Modification Reactions
The methacrylate double bond and fluorinated chain enable targeted modifications:
Esterification
HFMA reacts with carboxylic acids or anhydrides to form crosslinked networks. For example, reactions with succinic anhydride yield polyesters with enhanced solvent resistance .
Nucleophilic Substitution
The electron-withdrawing effect of fluorine atoms activates the carbonyl group for nucleophilic attack. Reactions with amines or thiols produce functionalized polymers for optoelectronic applications.
Research Findings
-
Surface Properties : HFMA-based polymers reduce surface energy to <20 mN/m , comparable to polytetrafluoroethylene (PTFE) .
-
Thermal Stability : Fluorine content correlates linearly with decomposition temperature () in copolymers .
-
Biomedical Compatibility : HFMA-modified hydrogels show reduced protein adsorption, making them suitable for antifouling coatings .
科学的研究の応用
Polymer Industry
Fluorinated Polymers:
HFMA is commonly used as a comonomer in the synthesis of fluorinated polymers such as polytetrafluoroethylene (PTFE), perfluoroalkoxy (PFA), and fluorinated ethylene propylene (FEP). These polymers benefit from enhanced thermal stability and chemical resistance due to the incorporation of HFMA .
Functional Polymers:
HFMA serves as a monomer for creating functional polymers including:
- Hydrogels: Used in biomedical applications due to improved biocompatibility.
- Block Copolymers: These materials exhibit unique properties like lubricity and fouling resistance.
- Graft Copolymers: Enhance performance in automotive and aerospace applications .
Optical Coatings
Polymers derived from HFMA are employed in photonics and optical coatings due to their unique refractive index properties. They are critical in developing high-performance optical devices .
Biomedical Applications
The biocompatibility and low surface energy of HFMA-based polymers make them suitable for medical devices and drug delivery systems. Their resistance to fouling is particularly beneficial in applications where biological interactions are critical .
Coatings and Membranes
Due to its chemical resistance and low surface energy properties, HFMA is used in coatings for various substrates to provide protective barriers against environmental factors. Additionally, membranes made from HFMA polymers are utilized in filtration processes and separation technologies .
Case Study 1: Development of Fluorinated Coatings
A study investigated the use of HFMA as a comonomer in developing fluorinated coatings that exhibit superior durability and chemical resistance compared to traditional coatings. The resulting materials showed enhanced performance in harsh environments commonly encountered in industrial applications.
Case Study 2: Biomedical Hydrogel Applications
Research focused on synthesizing hydrogels from HFMA for use in drug delivery systems. The hydrogels demonstrated improved release profiles and biocompatibility compared to non-fluorinated counterparts. This study highlights HFMA's potential in advancing biomedical technologies.
作用機序
The mechanism of action of 2,2,3,3,4,4,4-Heptafluorobutyl methacrylate primarily involves its ability to form strong, stable polymers. The fluorine atoms in the compound contribute to its low surface energy and high chemical resistance. These properties are crucial in applications requiring durable and long-lasting materials .
類似化合物との比較
- 2,2,3,3,4,4-Hexafluorobutyl methacrylate
- 2,2,2-Trifluoroethyl methacrylate
- 2,2,3,3,4,4,5,5-Octafluoropentyl methacrylate
Uniqueness: 2,2,3,3,4,4,4-Heptafluorobutyl methacrylate stands out due to its higher fluorine content, which enhances its thermal stability and chemical resistance compared to similar compounds .
生物活性
2,2,3,3,4,4,4-Heptafluorobutyl methacrylate (HFBA) is a fluorinated methacrylate compound that has gained attention for its unique properties and potential applications in various fields such as materials science and biomedical engineering. This article reviews the biological activity of HFBA, focusing on its synthesis, polymerization behavior, and biological interactions.
Synthesis and Polymerization
HFBA can be synthesized through various methods, including free radical polymerization and controlled radical polymerization techniques. One significant study utilized dibenzyl trithiocarbonate (DBTTC) as a chain transfer agent in the copolymerization of HFBA with other monomers like n-butyl acrylate (nBuA) in dimethylformamide (DMF) at elevated temperatures. The resulting copolymers exhibited well-defined structures and were characterized by nuclear magnetic resonance (NMR) spectroscopy and size exclusion chromatography (SEC) .
Table 1: Copolymer Characteristics
Copolymer Composition | Mn (NMR) g/mol | Mn (SEC) g/mol | PDI |
---|---|---|---|
Poly(nBuA-co-HFBA)1 | 1900 | 4100 | 1.36 |
Poly(nBuA-co-HFBA)2 | 4500 | 7300 | 1.26 |
Poly(nBuA-co-HFBA)3 | 6300 | 9600 | 1.23 |
Poly(nBuA-co-HFBA)4 | 7800 | 11300 | 1.25 |
Poly(nBuA-co-HFBA)5 | 39700 | 48700 | 1.27 |
Biological Activity
The biological activity of HFBA has been investigated primarily in the context of its use in dental materials and coatings. Research indicates that HFBA-modified materials exhibit enhanced antibacterial properties. For instance, a study demonstrated that dental composites incorporating HFBA showed reduced bacterial adhesion compared to traditional materials . This property is particularly valuable in preventing biofilm formation on dental surfaces.
Additionally, the incorporation of HFBA into polymeric matrices has been shown to influence cell adhesion and proliferation. The fluorinated nature of HFBA contributes to altered surface energy characteristics, which can enhance biocompatibility and reduce protein adsorption .
Case Studies
- Antibacterial Properties : A study evaluated the antibacterial efficacy of dental resin composites modified with HFBA. The results indicated a significant reduction in bacterial adhesion compared to controls without HFBA. This suggests potential applications in improving the longevity and performance of dental restoratives .
- Cellular Interactions : Another investigation focused on the effects of HFBA-containing polymers on human cell lines. The study found that these polymers supported cell viability and proliferation while maintaining lower levels of inflammatory cytokines compared to non-fluorinated counterparts .
Research Findings
- Fluorescence Imaging : Recent advancements in fluorescence imaging have utilized HFBA-based polymers for monitoring cellular processes. These polymers can be engineered to provide specific responses under fluorescent excitation, facilitating real-time imaging of cellular dynamics .
- Surface Modification : HFBA has been used to modify surfaces for improved biocompatibility. Studies have shown that surfaces treated with HFBA-containing polymers exhibit reduced fouling and enhanced interaction with biological systems .
特性
IUPAC Name |
2,2,3,3,4,4,4-heptafluorobutyl 2-methylprop-2-enoate | |
---|---|---|
Source | PubChem | |
URL | https://pubchem.ncbi.nlm.nih.gov | |
Description | Data deposited in or computed by PubChem | |
InChI |
InChI=1S/C8H7F7O2/c1-4(2)5(16)17-3-6(9,10)7(11,12)8(13,14)15/h1,3H2,2H3 | |
Source | PubChem | |
URL | https://pubchem.ncbi.nlm.nih.gov | |
Description | Data deposited in or computed by PubChem | |
InChI Key |
VIEHKBXCWMMOOU-UHFFFAOYSA-N | |
Source | PubChem | |
URL | https://pubchem.ncbi.nlm.nih.gov | |
Description | Data deposited in or computed by PubChem | |
Canonical SMILES |
CC(=C)C(=O)OCC(C(C(F)(F)F)(F)F)(F)F | |
Source | PubChem | |
URL | https://pubchem.ncbi.nlm.nih.gov | |
Description | Data deposited in or computed by PubChem | |
Molecular Formula |
C8H7F7O2 | |
Source | PubChem | |
URL | https://pubchem.ncbi.nlm.nih.gov | |
Description | Data deposited in or computed by PubChem | |
Related CAS |
31623-04-8 | |
Record name | 2-Propenoic acid, 2-methyl-, 2,2,3,3,4,4,4-heptafluorobutyl ester, homopolymer | |
Source | CAS Common Chemistry | |
URL | https://commonchemistry.cas.org/detail?cas_rn=31623-04-8 | |
Description | CAS Common Chemistry is an open community resource for accessing chemical information. Nearly 500,000 chemical substances from CAS REGISTRY cover areas of community interest, including common and frequently regulated chemicals, and those relevant to high school and undergraduate chemistry classes. This chemical information, curated by our expert scientists, is provided in alignment with our mission as a division of the American Chemical Society. | |
Explanation | The data from CAS Common Chemistry is provided under a CC-BY-NC 4.0 license, unless otherwise stated. | |
DSSTOX Substance ID |
DTXSID3065586 | |
Record name | (Perfluoropropyl)methyl methacrylate | |
Source | EPA DSSTox | |
URL | https://comptox.epa.gov/dashboard/DTXSID3065586 | |
Description | DSSTox provides a high quality public chemistry resource for supporting improved predictive toxicology. | |
Molecular Weight |
268.13 g/mol | |
Source | PubChem | |
URL | https://pubchem.ncbi.nlm.nih.gov | |
Description | Data deposited in or computed by PubChem | |
CAS No. |
13695-31-3 | |
Record name | 2,2,3,3,4,4,4-Heptafluorobutyl 2-methyl-2-propenoate | |
Source | CAS Common Chemistry | |
URL | https://commonchemistry.cas.org/detail?cas_rn=13695-31-3 | |
Description | CAS Common Chemistry is an open community resource for accessing chemical information. Nearly 500,000 chemical substances from CAS REGISTRY cover areas of community interest, including common and frequently regulated chemicals, and those relevant to high school and undergraduate chemistry classes. This chemical information, curated by our expert scientists, is provided in alignment with our mission as a division of the American Chemical Society. | |
Explanation | The data from CAS Common Chemistry is provided under a CC-BY-NC 4.0 license, unless otherwise stated. | |
Record name | Heptafluorobutyl methacrylate | |
Source | ChemIDplus | |
URL | https://pubchem.ncbi.nlm.nih.gov/substance/?source=chemidplus&sourceid=0013695313 | |
Description | ChemIDplus is a free, web search system that provides access to the structure and nomenclature authority files used for the identification of chemical substances cited in National Library of Medicine (NLM) databases, including the TOXNET system. | |
Record name | 2-Propenoic acid, 2-methyl-, 2,2,3,3,4,4,4-heptafluorobutyl ester | |
Source | EPA Chemicals under the TSCA | |
URL | https://www.epa.gov/chemicals-under-tsca | |
Description | EPA Chemicals under the Toxic Substances Control Act (TSCA) collection contains information on chemicals and their regulations under TSCA, including non-confidential content from the TSCA Chemical Substance Inventory and Chemical Data Reporting. | |
Record name | (Perfluoropropyl)methyl methacrylate | |
Source | EPA DSSTox | |
URL | https://comptox.epa.gov/dashboard/DTXSID3065586 | |
Description | DSSTox provides a high quality public chemistry resource for supporting improved predictive toxicology. | |
Record name | 2,2,3,3,4,4,4-heptafluorobutyl methacrylate | |
Source | European Chemicals Agency (ECHA) | |
URL | https://echa.europa.eu/substance-information/-/substanceinfo/100.033.819 | |
Description | The European Chemicals Agency (ECHA) is an agency of the European Union which is the driving force among regulatory authorities in implementing the EU's groundbreaking chemicals legislation for the benefit of human health and the environment as well as for innovation and competitiveness. | |
Explanation | Use of the information, documents and data from the ECHA website is subject to the terms and conditions of this Legal Notice, and subject to other binding limitations provided for under applicable law, the information, documents and data made available on the ECHA website may be reproduced, distributed and/or used, totally or in part, for non-commercial purposes provided that ECHA is acknowledged as the source: "Source: European Chemicals Agency, http://echa.europa.eu/". Such acknowledgement must be included in each copy of the material. ECHA permits and encourages organisations and individuals to create links to the ECHA website under the following cumulative conditions: Links can only be made to webpages that provide a link to the Legal Notice page. | |
Retrosynthesis Analysis
AI-Powered Synthesis Planning: Our tool employs the Template_relevance Pistachio, Template_relevance Bkms_metabolic, Template_relevance Pistachio_ringbreaker, Template_relevance Reaxys, Template_relevance Reaxys_biocatalysis model, leveraging a vast database of chemical reactions to predict feasible synthetic routes.
One-Step Synthesis Focus: Specifically designed for one-step synthesis, it provides concise and direct routes for your target compounds, streamlining the synthesis process.
Accurate Predictions: Utilizing the extensive PISTACHIO, BKMS_METABOLIC, PISTACHIO_RINGBREAKER, REAXYS, REAXYS_BIOCATALYSIS database, our tool offers high-accuracy predictions, reflecting the latest in chemical research and data.
Strategy Settings
Precursor scoring | Relevance Heuristic |
---|---|
Min. plausibility | 0.01 |
Model | Template_relevance |
Template Set | Pistachio/Bkms_metabolic/Pistachio_ringbreaker/Reaxys/Reaxys_biocatalysis |
Top-N result to add to graph | 6 |
Feasible Synthetic Routes
ANone: The molecular formula of HFBMA is C8H9F7O2, and its molecular weight is 272.15 g/mol.
A: Yes, various studies utilize spectroscopic techniques to characterize HFBMA. For instance, researchers employed Proton Nuclear Magnetic Resonance (1H NMR) spectroscopy and Fourier Transform Infrared (FTIR) spectroscopy to confirm the chemical structure and composition of HFBMA-containing polymers. [ [], [] ]
A: Studies show that adding HFBMA to fluorinated dimethacrylate (FDMA)/tricyclo (5.2.1.0) decanedimethanol diacrylate (SR833s) based dental resin composites significantly reduces surface free energy. [ [] ] This modification leads to improved anti-bacterial adhesion effects against Streptococcus mutans, making these composites promising for dental applications.
A: Incorporating HFBMA as a coil segment in polyfluorene-based rod-coil block copolymers can impact their spectral stability. While some coil segments like poly(butyl methacrylate) improve stability, HFBMA can lead to undesirable low-energy emission. [ [] ] Careful consideration of coil segment choice is crucial for tuning the optical properties of these materials.
A: Yes, researchers successfully grafted HFBMA onto polyethylene terephthalate (PET) ion-track membranes via photoinitiated graft polymerization. [ [], [] ] This modification increased the water contact angle, making the membranes hydrophobic and suitable for water desalination via membrane distillation.
A: Studies comparing statistical and block copolymers of methyl methacrylate (MMA) and HFBMA revealed that block copolymers consistently exhibited lower surface energies. [ [] ] This difference is attributed to the phase-separated domains formed in block copolymers, facilitating the presentation of fluorinated segments at the surface.
A: Research indicates that increasing fluorine content in methacrylate polymers generally leads to increased resistance to optical radiation damage. Specifically, poly(1H,1H-heptafluorobutyl methacrylate) showed superior UV/visible transmission after exposure to gamma radiation. [ [] ]
A: Studies on 1H, 1H, 3H-hexafluorobutyl and 1H, 1H-heptafluorobutyl methacrylate polymers revealed that they exhibit good gamma radiation stability. [ [] ] Poly(heptafluorobutyl methacrylate), in particular, showed remarkable resistance to radiation-induced discoloration and possessed the lowest refractive index among the tested compositions.
A: Yes, highly charged, monodisperse spherical particles with diameters ranging from 50 to 250 nm have been synthesized using HFBMA via emulsion polymerization. [ [] ] These particles, possessing a low refractive index, can form well-ordered crystalline colloidal arrays suitable for applications such as narrowband notch optical filters.
A: Researchers have incorporated HFBMA into oxygen sensor supports composed of poly(trimethylsilylmethyl methacrylate-co-1H, 1H-heptafluorobutyl methacrylate). [ [] ] By measuring oxygen diffusion coefficients, this study provides valuable insights into the relationship between polymer support properties and sensor response in luminescence-based oxygen sensors.
試験管内研究製品の免責事項と情報
BenchChemで提示されるすべての記事および製品情報は、情報提供を目的としています。BenchChemで購入可能な製品は、生体外研究のために特別に設計されています。生体外研究は、ラテン語の "in glass" に由来し、生物体の外で行われる実験を指します。これらの製品は医薬品または薬として分類されておらず、FDAから任何の医療状態、病気、または疾患の予防、治療、または治癒のために承認されていません。これらの製品を人間または動物に体内に導入する形態は、法律により厳格に禁止されています。これらのガイドラインに従うことは、研究と実験において法的および倫理的な基準の遵守を確実にするために重要です。