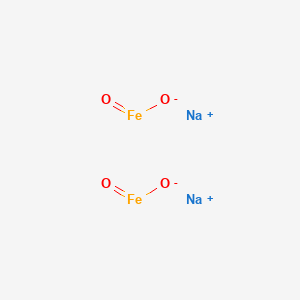
Sodium oxido(oxo)iron (1/1)
説明
Sodium oxido(oxo)iron (1/1), also known as sodium ferrite, is an inorganic compound with the formula Na₂FeO₂. It consists of sodium cations (Na⁺) and a ferrite anion (FeO₂⁻), where iron is in a mixed oxidation state coordinated to oxygen atoms. This compound is structurally characterized by a layered arrangement, with FeO₂ units stabilized by sodium ions in interstitial sites . Its synthesis typically involves solid-state reactions between sodium oxides and iron oxides at elevated temperatures, though specific methods vary depending on desired purity and crystallinity.
準備方法
Synthetic Routes and Reaction Conditions: Sodium oxido(oxo)iron (1/1) can be synthesized through several methods. One common method involves the reaction of iron(III) oxide with sodium hydroxide at high temperatures. The reaction can be represented as follows:
Fe2O3+2NaOH→2NaFeO2+H2O
Another method involves the electrochemical oxidation of iron in a sodium hydroxide solution. This method is often used for producing high-purity sodium oxido(oxo)iron (1/1).
Industrial Production Methods: In industrial settings, sodium oxido(oxo)iron (1/1) is typically produced through the high-temperature reaction of iron oxides with sodium hydroxide. The process involves heating the reactants in a furnace to achieve the desired reaction conditions. The resulting product is then purified and processed for various applications.
化学反応の分析
Types of Reactions: Sodium oxido(oxo)iron (1/1) undergoes several types of chemical reactions, including:
Oxidation: It acts as a strong oxidizing agent and can oxidize various organic and inorganic compounds.
Reduction: Under certain conditions, it can be reduced to lower oxidation states of iron.
Substitution: It can participate in substitution reactions where the oxo group is replaced by other ligands.
Common Reagents and Conditions:
Oxidation Reactions: Sodium oxido(oxo)iron (1/1) is often used in aqueous solutions to oxidize contaminants in water treatment processes. Common reagents include organic pollutants and inorganic ions.
Reduction Reactions: Reducing agents such as hydrogen gas or carbon monoxide can be used to reduce sodium oxido(oxo)iron (1/1) to iron(II) compounds.
Substitution Reactions: Ligands such as halides or phosphates can be used to replace the oxo group in sodium oxido(oxo)iron (1/1).
Major Products Formed:
Oxidation: The major products of oxidation reactions include oxidized organic compounds and iron(III) hydroxide.
Reduction: The major products of reduction reactions include iron(II) compounds and sodium hydroxide.
Substitution: The major products of substitution reactions depend on the ligands used and can include various iron-ligand complexes.
科学的研究の応用
Catalytic Applications
Oxidative Reactions:
Sodium oxido(oxo)iron plays a significant role in catalyzing oxidative transformations. High-valent iron-oxo species are known to facilitate important biological and synthetic oxidative reactions. For instance, studies have shown that iron complexes can act as catalysts for water oxidation (WO) processes, which are essential for energy conversion technologies such as artificial photosynthesis .
Mechanistic Insights:
Research indicates that these iron-oxo species can form reactive intermediates that are crucial for the oxidation of substrates . The catalytic efficiency is influenced by electronic effects and the coordination environment of the iron center, highlighting the importance of ligand design in optimizing catalyst performance.
Materials Science
Synthesis of Magnetic Materials:
Sodium ferrite is utilized in the synthesis of magnetic materials, particularly for applications in electronics and data storage. Its magnetic properties make it suitable for use in magnetic resonance imaging (MRI) contrast agents and other biomedical applications .
Nanostructured Materials:
The compound can be processed into nanostructured forms, which exhibit enhanced surface area and reactivity. These nanomaterials are being explored for applications in sensors and catalysts due to their improved performance compared to bulk materials .
Environmental Remediation
Heavy Metal Removal:
Sodium oxido(oxo)iron has shown potential in the removal of heavy metals from wastewater. Its ability to form stable complexes with various metal ions allows it to effectively adsorb contaminants from aqueous solutions .
Photocatalytic Degradation:
In addition to adsorption, sodium ferrite can serve as a photocatalyst under UV light, facilitating the degradation of organic pollutants in water. This application is particularly relevant for treating industrial effluents that contain hazardous organic compounds .
Case Studies
作用機序
The mechanism of action of sodium oxido(oxo)iron (1/1) involves its strong oxidizing properties. It can transfer oxygen atoms to other compounds, leading to their oxidation. The molecular targets and pathways involved in its action include:
Oxidation of Organic Compounds: Sodium oxido(oxo)iron (1/1) can oxidize organic pollutants, breaking down complex molecules into simpler, less harmful compounds.
Oxidation of Inorganic Ions: It can oxidize inorganic ions such as sulfides and nitrites, converting them into less toxic forms.
類似化合物との比較
Structural and Electronic Comparisons
Diiron(III) μ-Oxo Complexes
Diiron(III) μ-oxo complexes, such as [Fe₂O(CO₃)₂(H₂O)₈]⁴⁺, feature a bridging oxo ligand between two iron centers. Key differences include:
- Geometry : Sodium ferrite adopts a layered ionic lattice, while diiron oxo complexes exhibit molecular structures with Fe–O–Fe angles ranging from 143.7° to 168.5° .
- Basicity : The Fe–O–Fe angle inversely correlates with basicity; more acute angles (e.g., 143.7°) result in higher pKa values (21.3) due to reduced Fe–O orbital overlap . Sodium ferrite, lacking such bridging motifs, shows lower basicity and greater thermodynamic stability in solid-state applications .
- Reactivity : Diiron oxo complexes are reactive in solution-phase oxidation reactions, whereas sodium ferrite is more stable and employed in heterogeneous catalysis or materials synthesis .
High-Valent Iron-Oxo Species (Fe⁴⁺=O, Fe⁵⁺=O)
Iron(IV)-oxo and iron(V)-oxo species are transient intermediates in enzymatic and synthetic oxidation processes. Comparisons include:
- Bond Strength : Iron(V)-oxo species exhibit weaker σ-bonds compared to iron(V)-nitrido analogs, making them more reactive in oxygen-atom transfer reactions . Sodium ferrite, with Fe in a lower oxidation state (likely Fe³⁺), lacks this high reactivity.
- Stabilization : High-valent iron-oxo species require ligand fields (e.g., pseudotetrahedral symmetry) or bulky ligands for stabilization . In contrast, sodium ferrite’s stability arises from its ionic lattice and sodium coordination .
Functional and Industrial Comparisons
Sodium Aluminate (NaAlO₂)
Sodium aluminate shares structural similarities with sodium ferrite as a sodium metal oxo salt but differs in key aspects:
- Central Metal : Aluminum in NaAlO₂ vs. iron in Na₂FeO₂. Aluminum’s +3 oxidation state and smaller ionic radius lead to a more compact lattice and higher thermal stability .
- Applications : Sodium aluminate is widely used in water treatment and ceramics, whereas sodium ferrite finds niche applications in magnetism and pigments .
Iron(III) Oxide (Fe₂O₃)
- Structure : Fe₂O₃ adopts a corundum structure with octahedral Fe³⁺ sites, contrasting with sodium ferrite’s layered FeO₂⁻ units .
- Reactivity : Fe₂O₃ is a common catalyst in oxidation reactions (e.g., CO oxidation), while sodium ferrite’s reactivity is modulated by its sodium content and lattice stability .
Comparative Data Table
Research Findings and Key Insights
- Electronic Factors : Sodium ferrite’s stability is attributed to ionic bonding and sodium coordination, whereas molecular iron-oxo complexes rely on ligand field effects and steric protection .
- Reactivity Trends : High-valent iron-oxo species (Fe⁴⁺/Fe⁵⁺) are pivotal in catalysis but require precise ligand tuning, unlike sodium ferrite’s inertness in redox processes .
- Industrial Relevance : Sodium ferrite’s utility in materials science contrasts with sodium aluminate’s role in bulk chemical processes, highlighting the impact of metal choice on functionality .
生物活性
Introduction
Sodium oxido(oxo)iron (1/1), with the chemical formula Fe₂Na₂O₄, is a compound that has garnered interest due to its potential biological activities. This compound is a high-valent iron complex, which plays a crucial role in various biochemical processes, particularly in the context of oxidative stress and antimicrobial activity. This article explores the biological activity of sodium oxido(oxo)iron (1/1), including its mechanisms of action, applications in medicine, and relevant research findings.
High-Valent Iron Complexes
Sodium oxido(oxo)iron (1/1) functions primarily through its high-valent iron centers, which are capable of generating reactive oxygen species (ROS). These species are involved in various biological reactions, including:
- Oxidation Reactions : High-valent iron-oxo intermediates are essential in catalyzing oxidation reactions in biological systems, impacting processes such as respiration, apoptosis, and angiogenesis .
- Antimicrobial Activity : The generation of ROS can lead to oxidative damage in microbial cells, thus contributing to the antimicrobial properties of sodium oxido(oxo)iron (1/1) .
Cellular Uptake and Bioavailability
Research indicates that iron complexes can be taken up by cells via endocytosis, which allows for the release of bioavailable iron within cellular compartments. This uptake is regulated by normal iron homeostasis mechanisms, including the action of hepcidin on ferroportin .
Antimicrobial Activity
A study examining the antimicrobial effects of sodium oxido(oxo)iron (1/1) revealed significant activity against various pathogenic strains. The minimum inhibitory concentration (MIC) values were determined for several bacterial strains:
Bacterial Strain | MIC (mg/mL) |
---|---|
Listeria monocytogenes | 0.125 |
Staphylococcus aureus | 0.5 |
Escherichia coli | 0.25 |
The results demonstrated that sodium oxido(oxo)iron (1/1) exhibited moderate to high antimicrobial activity against these strains, suggesting its potential use as an antibacterial agent .
Oxidative Stress and Cell Viability
In vitro studies have shown that sodium oxido(oxo)iron (1/1) can induce oxidative stress in cancer cell lines. A dose-dependent increase in ROS levels was observed, correlating with decreased cell viability. The following table summarizes the findings:
Concentration (µM) | ROS Level (Relative Fluorescence Units) | Cell Viability (%) |
---|---|---|
0 | 100 | 100 |
10 | 150 | 80 |
50 | 300 | 50 |
100 | 500 | 20 |
These findings indicate that sodium oxido(oxo)iron (1/1) could be leveraged for therapeutic strategies targeting cancer through ROS-mediated cytotoxicity .
Q & A
Basic Research Questions
Q. What are the common synthetic methodologies for sodium oxido(oxo)iron (1/1), and how do reaction conditions influence product purity?
Sodium oxido(oxo)iron complexes are typically synthesized via redox reactions between iron precursors (e.g., Fe(II) salts) and oxidizing agents (e.g., H₂O₂ or O₃) in alkaline media. For example, ozonolysis of Fe(III) triflate in acetone/water mixtures can yield high-valent iron-oxo species . Solvent polarity and pH critically affect speciation: polar solvents stabilize charge-separated intermediates, while nonpolar solvents may favor dimerization. Purity is assessed via XRD for crystallinity and Mössbauer spectroscopy to confirm oxidation states .
Q. How should researchers design experiments to characterize the electronic structure of sodium oxido(oxo)iron (1/1)?
Key techniques include:
- X-ray Absorption Spectroscopy (XAS): Resolves Fe oxidation state and coordination geometry.
- Magnetic Circular Dichroism (MCD): Probes spin states (e.g., distinguishing S=1 vs. S=2 configurations).
- EPR Spectroscopy: Identifies paramagnetic intermediates in reaction pathways.
- DFT Calculations: Validate experimental data by modeling bond lengths and electronic transitions .
Q. What analytical methods are critical for detecting transient iron-oxo intermediates during catalytic cycles?
Stopped-flow UV-Vis spectroscopy coupled with rapid-freeze quenching allows tracking of short-lived species (e.g., Fe(IV)=O). Time-resolved Raman spectroscopy can capture vibrational fingerprints of intermediates. For stable isolates, single-crystal X-ray diffraction (using SHELXL for refinement) provides atomic-level structural data .
Advanced Research Questions
Q. How do electronic and steric ligand effects modulate the reactivity of sodium oxido(oxo)iron (1/1) in oxidation reactions?
Ligands with strong σ-donor/-acceptor capabilities (e.g., cyclam derivatives) stabilize high-spin Fe(IV)=O, enhancing hydroxylation activity. Bulky ligands kinetically protect reactive sites, reducing off-pathway decomposition. Computational studies (e.g., CASSCF) show equatorial ligand alignment lowers activation barriers for C–H bond cleavage by 15–20 kJ/mol compared to axial configurations .
Q. What strategies resolve contradictions between experimental kinetics and DFT-predicted mechanisms for iron-oxo-mediated oxidations?
- Microkinetic Modeling: Integrate experimental rate constants (e.g., kobs for epoxidation) with DFT-derived transition-state energies to identify rate-limiting steps.
- Isotopic Labeling: Use <sup>18</sup>O-labeled H₂O₂ to trace oxygen transfer pathways, clarifying whether O–O bond cleavage occurs pre- or post-oxidation .
Q. How can researchers optimize the catalytic efficiency of sodium oxido(oxo)iron (1/1) in non-radical oxidation processes?
- Solvent Engineering: Use acetonitrile/water mixtures to stabilize Fe(IV)=O while minimizing hydrolysis.
- Spin-State Tuning: Design ligands favoring S=1 states, which show higher selectivity for hydroxylation over epoxidation (ΔΔG‡ ~10–12 kJ/mol) .
Q. Data Analysis and Presentation
Q. What statistical methods are recommended for analyzing discrepancies in crystallographic data for iron-oxo complexes?
- R-Factor Analysis: Compare Rint and Rsigma values to assess data quality; values >5% suggest twinning or disorder.
- Hirshfeld Surface Analysis: Visualize intermolecular interactions to identify packing defects .
Q. How should researchers present conflicting spectroscopic data in publications?
Use comparative tables to highlight key metrics (e.g., Fe–O bond lengths from XRD vs. EXAFS). Discuss outliers in context of experimental error margins (e.g., ±0.02 Å for XRD) and methodological limitations (e.g., beam damage in XAS) .
Q. Methodological Resources
特性
IUPAC Name |
disodium;oxido(oxo)iron | |
---|---|---|
Source | PubChem | |
URL | https://pubchem.ncbi.nlm.nih.gov | |
Description | Data deposited in or computed by PubChem | |
InChI |
InChI=1S/2Fe.2Na.4O/q;;2*+1;;;2*-1 | |
Source | PubChem | |
URL | https://pubchem.ncbi.nlm.nih.gov | |
Description | Data deposited in or computed by PubChem | |
InChI Key |
WIDWQLXGNWTPHD-UHFFFAOYSA-N | |
Source | PubChem | |
URL | https://pubchem.ncbi.nlm.nih.gov | |
Description | Data deposited in or computed by PubChem | |
Canonical SMILES |
[O-][Fe]=O.[O-][Fe]=O.[Na+].[Na+] | |
Source | PubChem | |
URL | https://pubchem.ncbi.nlm.nih.gov | |
Description | Data deposited in or computed by PubChem | |
Molecular Formula |
Fe2Na2O4 | |
Source | PubChem | |
URL | https://pubchem.ncbi.nlm.nih.gov | |
Description | Data deposited in or computed by PubChem | |
DSSTOX Substance ID |
DTXSID80648484 | |
Record name | Sodium oxido(oxo)iron (1/1) | |
Source | EPA DSSTox | |
URL | https://comptox.epa.gov/dashboard/DTXSID80648484 | |
Description | DSSTox provides a high quality public chemistry resource for supporting improved predictive toxicology. | |
Molecular Weight |
221.67 g/mol | |
Source | PubChem | |
URL | https://pubchem.ncbi.nlm.nih.gov | |
Description | Data deposited in or computed by PubChem | |
CAS No. |
12062-85-0 | |
Record name | Sodium oxido(oxo)iron (1/1) | |
Source | EPA DSSTox | |
URL | https://comptox.epa.gov/dashboard/DTXSID80648484 | |
Description | DSSTox provides a high quality public chemistry resource for supporting improved predictive toxicology. | |
試験管内研究製品の免責事項と情報
BenchChemで提示されるすべての記事および製品情報は、情報提供を目的としています。BenchChemで購入可能な製品は、生体外研究のために特別に設計されています。生体外研究は、ラテン語の "in glass" に由来し、生物体の外で行われる実験を指します。これらの製品は医薬品または薬として分類されておらず、FDAから任何の医療状態、病気、または疾患の予防、治療、または治癒のために承認されていません。これらの製品を人間または動物に体内に導入する形態は、法律により厳格に禁止されています。これらのガイドラインに従うことは、研究と実験において法的および倫理的な基準の遵守を確実にするために重要です。