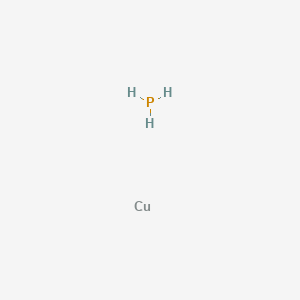
銅ホスフィン (1/1)
説明
Copper–phosphane (1/1) is a coordination compound consisting of copper and phosphane in a 1:1 ratio. This compound is notable for its applications in various fields, including catalysis, materials science, and medicinal chemistry. The molecular formula for Copper–phosphane (1/1) is CuH₃P, and it has a molecular weight of 97.54 g/mol.
科学的研究の応用
Copper–phosphane (1/1) has a wide range of applications in scientific research:
Chemistry: Used as a catalyst in organic synthesis, particularly in cross-coupling reactions and hydrogenation processes.
Biology: Investigated for its role in biological systems, including its potential as an antimicrobial agent.
Medicine: Explored for its anticancer properties, where it facilitates the accumulation of copper in cancer cells, leading to cytotoxic effects.
Industry: Utilized in the production of advanced materials, including conductive polymers and nanomaterials.
準備方法
Synthetic Routes and Reaction Conditions: Copper–phosphane (1/1) can be synthesized through several methods, including:
Direct Combination: Copper metal reacts with phosphane gas under controlled conditions to form the compound.
Solution-Based Synthesis: Copper salts (such as copper(II) sulfate) react with phosphane in a solvent like ethanol or acetonitrile, often in the presence of a reducing agent to facilitate the formation of the desired product.
Industrial Production Methods: In industrial settings, the production of Copper–phosphane (1/1) typically involves:
Bulk Reaction: Large-scale reactors where copper and phosphane are combined under high pressure and temperature to ensure complete reaction.
Purification: The crude product is purified using techniques such as recrystallization or chromatography to obtain high-purity Copper–phosphane (1/1).
Types of Reactions:
Oxidation: Copper–phosphane (1/1) can undergo oxidation reactions, where the copper center is oxidized, often leading to the formation of copper(II) complexes.
Reduction: The compound can be reduced back to its elemental forms under specific conditions.
Substitution: Ligand substitution reactions are common, where the phosphane ligand can be replaced by other ligands such as amines or phosphites.
Common Reagents and Conditions:
Oxidizing Agents: Hydrogen peroxide, oxygen, or other peroxides.
Reducing Agents: Sodium borohydride, lithium aluminum hydride.
Solvents: Ethanol, acetonitrile, and other polar solvents.
Major Products:
Oxidation Products: Copper(II) phosphane complexes.
Reduction Products: Elemental copper and free phosphane.
Substitution Products: Copper complexes with different ligands.
作用機序
The mechanism by which Copper–phosphane (1/1) exerts its effects involves:
Molecular Targets: The copper center interacts with various biological molecules, including proteins and nucleic acids.
Pathways Involved: Copper–phosphane (1/1) can generate reactive oxygen species (ROS) in cells, leading to oxidative stress and cell death in cancer cells. It also participates in electron transfer reactions, which are crucial in catalytic processes.
類似化合物との比較
Copper(I) chloride (CuCl): Similar in terms of copper oxidation state but lacks the phosphane ligand.
Copper(II) sulfate (CuSO₄): A common copper compound with different chemical properties and applications.
Triphenylphosphine (PPh₃): A phosphane compound without copper, used widely in organic synthesis.
Uniqueness: Copper–phosphane (1/1) is unique due to its specific coordination chemistry, which allows it to participate in a variety of chemical reactions and applications that are not possible with other copper or phosphane compounds. Its ability to act as a catalyst and its potential biological activity make it a compound of significant interest in both research and industrial applications.
生物活性
Copper-phosphane complexes, particularly those with a 1:1 stoichiometry, have garnered significant attention in the field of medicinal chemistry due to their diverse biological activities. This article explores the biological activity of these complexes, focusing on their anticancer properties, mechanisms of action, and potential therapeutic applications.
Overview of Copper's Biological Role
Copper is an essential trace element in biological systems, playing a crucial role in various enzymatic processes. It is involved in electron transport, redox reactions, and the formation of reactive oxygen species (ROS), which can induce apoptosis in cancer cells. Copper's ability to exist in multiple oxidation states (Cu(I) and Cu(II)) allows it to participate in diverse biochemical pathways, making copper-based compounds attractive for therapeutic use .
Copper-phosphane complexes exhibit several mechanisms that contribute to their biological activity:
- Induction of Apoptosis : These complexes can activate apoptosis signaling pathways through ROS generation. The oxidative stress induced by copper ions leads to DNA damage and subsequent cell death .
- Inhibition of Angiogenesis : By interfering with the vascular endothelial growth factor (VEGF) signaling pathways, copper complexes can inhibit the formation of new blood vessels, which is critical for tumor growth .
- Targeting DNA : Copper complexes can bind to DNA and inhibit topoisomerases, enzymes essential for DNA replication and repair. This interaction can lead to DNA strand breaks and cell cycle arrest .
- Antimicrobial Activity : Some copper-phosphane complexes have demonstrated significant antimicrobial properties against various pathogens, suggesting potential applications in treating infections .
Anticancer Activity
Recent studies have highlighted the anticancer potential of copper-phosphane complexes:
- Machado et al. (2023) investigated copper(I) complexes containing phosphane ligands and reported dual anticancer and antiparasitic properties. These complexes exhibited cytotoxic effects against chemoresistant prostate cancer cells (PC3) and were effectively taken up by ovarian cancer cells (OVCAR3). The study emphasized the role of apoptosis in mediating cell death .
- Abdolmaleki et al. (2023) demonstrated that a specific copper(II) complex induced apoptosis in HeLa cells through a caspase-dependent pathway. The complex showed strong cytotoxicity compared to its ligands, indicating that coordination with copper enhances biological activity .
- Beckford and Webb (2017) reported that certain copper-phosphane complexes exhibited potent inhibitory effects on topoisomerases, with dissociation constants indicating strong binding affinity to DNA. This property is crucial for developing effective anticancer agents .
Antimicrobial Properties
Copper-phosphane complexes also show promise as antimicrobial agents:
- A comparative study revealed that copper complexes had minimum inhibitory concentration (MIC) values significantly lower than those of zinc complexes against both Gram-positive and Gram-negative bacteria. This suggests a higher efficacy for copper-based compounds in microbial inhibition .
Data Summary
The following table summarizes key findings related to the biological activity of copper-phosphane (1/1) complexes:
Study | Complex Type | Biological Activity | Key Findings |
---|---|---|---|
Machado et al. (2023) | Copper(I)-Phosphane | Anticancer & Antiparasitic | Induced apoptosis in OVCAR3 & PC3 cells |
Abdolmaleki et al. (2023) | Copper(II) Complex | Anticancer | Strong cytotoxicity via caspase-dependent pathway |
Beckford & Webb (2017) | Copper-Phosphane | DNA Interaction | Inhibitory effects on topoisomerases with strong binding affinity |
Comparative Study | Copper Complexes | Antimicrobial | Lower MIC values than zinc complexes against pathogens |
特性
IUPAC Name |
copper;phosphane | |
---|---|---|
Source | PubChem | |
URL | https://pubchem.ncbi.nlm.nih.gov | |
Description | Data deposited in or computed by PubChem | |
InChI |
InChI=1S/Cu.H3P/h;1H3 | |
Source | PubChem | |
URL | https://pubchem.ncbi.nlm.nih.gov | |
Description | Data deposited in or computed by PubChem | |
InChI Key |
RRMIKQFUEXQAQP-UHFFFAOYSA-N | |
Source | PubChem | |
URL | https://pubchem.ncbi.nlm.nih.gov | |
Description | Data deposited in or computed by PubChem | |
Canonical SMILES |
P.[Cu] | |
Source | PubChem | |
URL | https://pubchem.ncbi.nlm.nih.gov | |
Description | Data deposited in or computed by PubChem | |
Molecular Formula |
CuH3P | |
Source | PubChem | |
URL | https://pubchem.ncbi.nlm.nih.gov | |
Description | Data deposited in or computed by PubChem | |
DSSTOX Substance ID |
DTXSID10648730 | |
Record name | Copper--phosphane (1/1) | |
Source | EPA DSSTox | |
URL | https://comptox.epa.gov/dashboard/DTXSID10648730 | |
Description | DSSTox provides a high quality public chemistry resource for supporting improved predictive toxicology. | |
Molecular Weight |
97.54 g/mol | |
Source | PubChem | |
URL | https://pubchem.ncbi.nlm.nih.gov | |
Description | Data deposited in or computed by PubChem | |
CAS No. |
12517-41-8 | |
Record name | Copper--phosphane (1/1) | |
Source | EPA DSSTox | |
URL | https://comptox.epa.gov/dashboard/DTXSID10648730 | |
Description | DSSTox provides a high quality public chemistry resource for supporting improved predictive toxicology. | |
Q1: What is unique about the copper complexes described in this research and how does their structure relate to their water solubility?
A1: The research describes the synthesis and characterization of two novel copper(I) complexes: 5 (1) and (2), where PTAH is the N-protonated form of 1,3,5-triaza-7-phosphaadamantane (PTA). [] These compounds represent the first examples of copper complexes containing the PTA ligand, which possesses a cage-like structure. [] Both complexes exhibit a tetrahedral coordination geometry around the copper center, with four PTAH/PTA ligands bound through their phosphorus atoms. [] The presence of multiple PTA ligands, coupled with the ionic nature of the complexes due to the nitrate counterions, contributes significantly to their remarkable water solubility, a feature not commonly observed in copper phosphane complexes. []
試験管内研究製品の免責事項と情報
BenchChemで提示されるすべての記事および製品情報は、情報提供を目的としています。BenchChemで購入可能な製品は、生体外研究のために特別に設計されています。生体外研究は、ラテン語の "in glass" に由来し、生物体の外で行われる実験を指します。これらの製品は医薬品または薬として分類されておらず、FDAから任何の医療状態、病気、または疾患の予防、治療、または治癒のために承認されていません。これらの製品を人間または動物に体内に導入する形態は、法律により厳格に禁止されています。これらのガイドラインに従うことは、研究と実験において法的および倫理的な基準の遵守を確実にするために重要です。