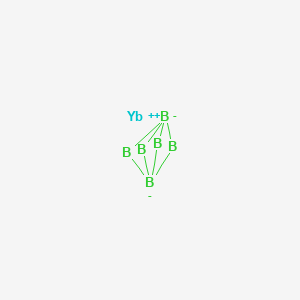
Ytterbium boride
説明
Ytterbium boride encompasses a family of rare-earth borides, including ytterbium diboride (YbB₂), tetraboride (YbB₄), and hexaboride (YbB₆). These compounds exhibit distinct crystallographic and electronic properties, making them relevant for thermoelectric, magnetic, and high-temperature applications.
Crystal Structures:
- YbB₂ adopts the simple AlB₂-type hexagonal structure (space group P6/mmm), where boron forms a planar hexagonal network sandwiched between ytterbium layers .
- YbB₄ and YbB₆ feature more complex boron frameworks. YbB₆ has a cubic CsCl-type structure (space group Pm-3m), with octahedral boron clusters forming a rigid 3D network .
Synthesis:
Thin films of ytterbium borides are synthesized via hybrid physical–chemical vapor deposition (HPCVD) using decaborane (B₁₀H₁₄) as a boron precursor. Growth occurs at 800–1,050°C on MgO substrates, achieving high phase purity and growth rates ~10× faster than conventional methods .Properties:
特性
IUPAC Name |
2,3,5,6-tetrabora-1,4-diboranuidapentacyclo[3.1.0.01,3.02,4.04,6]hexane;ytterbium(2+) | |
---|---|---|
Source | PubChem | |
URL | https://pubchem.ncbi.nlm.nih.gov | |
Description | Data deposited in or computed by PubChem | |
InChI |
InChI=1S/B6.Yb/c1-2-5(1)3-4(5)6(1,2)3;/q-2;+2 | |
Source | PubChem | |
URL | https://pubchem.ncbi.nlm.nih.gov | |
Description | Data deposited in or computed by PubChem | |
InChI Key |
VDVYOCSKBADDSK-UHFFFAOYSA-N | |
Source | PubChem | |
URL | https://pubchem.ncbi.nlm.nih.gov | |
Description | Data deposited in or computed by PubChem | |
Canonical SMILES |
B12B3[B-]14B5[B-]23B45.[Yb+2] | |
Source | PubChem | |
URL | https://pubchem.ncbi.nlm.nih.gov | |
Description | Data deposited in or computed by PubChem | |
Molecular Formula |
B6Yb | |
Source | PubChem | |
URL | https://pubchem.ncbi.nlm.nih.gov | |
Description | Data deposited in or computed by PubChem | |
Molecular Weight |
237.9 g/mol | |
Source | PubChem | |
URL | https://pubchem.ncbi.nlm.nih.gov | |
Description | Data deposited in or computed by PubChem | |
CAS No. |
12008-33-2 | |
Record name | Ytterbium boride (YbB6), (OC-6-11)- | |
Source | EPA Chemicals under the TSCA | |
URL | https://www.epa.gov/chemicals-under-tsca | |
Description | EPA Chemicals under the Toxic Substances Control Act (TSCA) collection contains information on chemicals and their regulations under TSCA, including non-confidential content from the TSCA Chemical Substance Inventory and Chemical Data Reporting. | |
Record name | Ytterbium hexaboride | |
Source | European Chemicals Agency (ECHA) | |
URL | https://echa.europa.eu/substance-information/-/substanceinfo/100.031.387 | |
Description | The European Chemicals Agency (ECHA) is an agency of the European Union which is the driving force among regulatory authorities in implementing the EU's groundbreaking chemicals legislation for the benefit of human health and the environment as well as for innovation and competitiveness. | |
Explanation | Use of the information, documents and data from the ECHA website is subject to the terms and conditions of this Legal Notice, and subject to other binding limitations provided for under applicable law, the information, documents and data made available on the ECHA website may be reproduced, distributed and/or used, totally or in part, for non-commercial purposes provided that ECHA is acknowledged as the source: "Source: European Chemicals Agency, http://echa.europa.eu/". Such acknowledgement must be included in each copy of the material. ECHA permits and encourages organisations and individuals to create links to the ECHA website under the following cumulative conditions: Links can only be made to webpages that provide a link to the Legal Notice page. | |
準備方法
Solid-State Synthesis via Argon-Arc Melting and Annealing
Solid-state synthesis remains the most widely used method for producing bulk ytterbium boride compounds. This technique involves direct reaction of elemental ytterbium, boron, and optional transition metals under controlled atmospheres.
Argon-Arc Melting and Homogenization
Ytterbium borides such as YbPtB are synthesized by argon-arc melting stoichiometric mixtures of ytterbium (Yb), platinum (Pt), and boron (B) at temperatures exceeding 1,500°C. The molten alloy is rapidly cooled to room temperature under argon to prevent oxidation . Post-melting annealing at 780°C for 720 hours ensures homogenization and stabilizes the desired crystal phases. For example, annealing Yb-Pt-B mixtures yields single-phase YbPtB with a boron-deficient structure .
Key Parameters:
-
Elemental purity: ≥99.9% for Yb, B, and Pt.
-
Atmosphere: High-purity argon (≤1 ppm O).
-
Annealing duration: 240–720 hours.
Spark Plasma Sintering (SPS) with Flux Additives
While primarily used for yttrium aluminoborides, spark plasma sintering (SPS) with AlF flux offers a promising route for dense this compound ceramics. The flux enhances reactivity by forming a liquid phase during sintering, reducing secondary phases like YbB or YbB. A typical protocol involves:
-
Mixing Yb, B, and 5 wt% AlF.
-
Sintering at 1,773 K under 80 MPa uniaxial pressure for 5–60 minutes .
-
Post-sintering etching in NaOH to remove residual flux.
This method achieves 89–95% relative density in Yb-Al-B systems, though boron loss remains a challenge .
Vapor-Phase Deposition of Thin Films
Hybrid Physical-Chemical Vapor Deposition (HPCVD)
HPCVD enables epitaxial growth of this compound thin films for thermoelectric applications. The process combines physical vapor deposition of ytterbium with chemical vapor delivery of boron hydrides (e.g., BH).
Growth Conditions for YbB Thin Films :
Parameter | Value |
---|---|
Substrate | MgO (100) |
Growth temperature | 800–1,000°C |
Yb evaporation rate | 0.1–0.3 Å/s |
BH flow | 10–50 sccm |
Chamber pressure | 10–10 Torr |
Films grown under these conditions exhibit <5% lattice mismatch and high phase purity, as confirmed by X-ray diffraction (XRD) and reflection high-energy electron diffraction (RHEED) .
Phase Purity and Crystallinity Control
Achieving single-phase YbB requires precise control of boron-to-ytterbium ratios. Excess boron (>66 at%) promotes secondary phases like YbB, while boron deficiency (<50 at%) leads to Yb-rich impurities . In-situ RHEED monitoring during HPCVD allows real-time adjustment of deposition rates to maintain stoichiometry.
Structural and Phase Analysis of Synthesized Materials
X-ray Diffraction (XRD) Characterization
Rietveld refinement of XRD data reveals that argon-arc melted YbPtB adopts a tetragonal antiprismatic boron framework with Pt atoms occupying interstitial sites. The unit cell parameters expand linearly with Pt content (e.g., a = 7.21 Å to 7.35 Å for x = 1.0–1.34) .
Crystallographic Data for YbPtB:
-
Space group: I4/mmm
-
Lattice parameters: a = 7.35 Å, c = 4.12 Å
-
Density: 6.78 g/cm
Scanning Electron Microscopy (SEM) and Compositional Mapping
SEM-EDX analysis of spark plasma-sintered Yb-B samples shows AlO impurities (≤5 vol%) originating from oxygen contamination during milling . HPCVD-grown films exhibit columnar grain structures with 50–100 nm grain sizes , ideal for minimizing thermal conductivity in thermoelectrics .
Comparative Evaluation of Synthesis Methods
Table 1: Comparison of this compound Preparation Techniques
Method | Temperature Range | Time | Density | Purity | Application |
---|---|---|---|---|---|
Argon-arc melting | 1,500–1,800°C | 240–720 h | 70–85% | 90–95% | Bulk superconductors |
Spark plasma sintering | 1,500–1,773 K | 5–60 min | 85–95% | 80–90% | High-density ceramics |
HPCVD | 800–1,000°C | 1–4 h | 95–98% | ≥98% | Thin-film devices |
Solid-state methods excel in producing bulk materials but struggle with slow kinetics and impurity incorporation. Conversely, HPCVD offers superior purity and nanostructural control at the expense of scalability.
化学反応の分析
Types of Reactions: Ytterbium boride undergoes various chemical reactions, including oxidation, reduction, and substitution reactions.
Common Reagents and Conditions:
Oxidation: this compound can be oxidized in the presence of oxygen at elevated temperatures, forming ytterbium oxide and boron oxide.
Reduction: Reduction reactions typically involve the use of hydrogen or other reducing agents to convert this compound back to its elemental form.
Substitution: Substitution reactions can occur when this compound reacts with halogens, forming ytterbium halides and boron halides.
Major Products: The major products formed from these reactions include ytterbium oxide, boron oxide, ytterbium halides, and boron halides.
科学的研究の応用
Thermoelectric Applications
Ytterbium borides, particularly YbB2 and YbB6, exhibit promising thermoelectric properties, making them suitable for energy conversion applications. Thermoelectric materials can convert temperature differences into electrical voltage, which is crucial for waste heat recovery systems.
- Thermoelectric Properties : Research indicates that YbB thin films can achieve high thermoelectric performance due to their low thermal conductivity and high Seebeck coefficients. These properties are essential for maximizing the efficiency of thermoelectric generators (TEGs) .
- Synthesis Methods : The hybrid physical-chemical vapor deposition (HPCVD) technique has been employed to produce YbB thin films rapidly. This method allows for precise control over the growth conditions, leading to enhanced thermoelectric performance .
Material Science and Engineering
This compound is utilized in various advanced material applications due to its mechanical stability and thermal resistance.
- High-Temperature Stability : YbB exhibits exceptional thermal stability, making it suitable for use in high-temperature environments (above 1200 K). This characteristic is particularly valuable in aerospace and nuclear applications where materials must withstand extreme conditions .
- Refractory Ceramics : As a refractory material, this compound can be used in the production of ceramics that require high durability and thermal resistance. Its mechanical properties make it a candidate for components subjected to severe wear and tear .
Quantum Computing
Ytterbium ions, especially the isotope , are being explored for their potential in quantum computing.
- Qubit Development : The stable electronic states of ytterbium ions allow them to function effectively as qubits, the fundamental units of quantum information. This stability is crucial for maintaining coherence times necessary for quantum computations .
- Applications in Quantum Technologies : The use of ytterbium ions in quantum computing could revolutionize fields such as cryptography and complex data analysis by enabling faster processing speeds compared to classical computers .
Catalytic Applications
Ytterbium compounds also serve as effective catalysts in various chemical reactions.
- Industrial Catalysis : Ytterbium borides are used as catalysts in the synthesis of plastics and pharmaceuticals. Their ability to enhance reaction rates while reducing energy consumption makes them attractive for industrial applications .
Summary Table of Applications
Application Area | Description | Key Benefits |
---|---|---|
Thermoelectrics | Energy conversion from heat to electricity | High efficiency, low thermal conductivity |
Material Science | High-temperature stable refractory ceramics | Mechanical durability |
Quantum Computing | Use of ytterbium ions as qubits | Enhanced processing speeds |
Catalysis | Catalysts in chemical synthesis | Reduced energy consumption |
Case Studies and Research Findings
- Thermoelectric Performance : A study on YbB thin films demonstrated a significant enhancement in thermoelectric efficiency due to optimized growth conditions via HPCVD, achieving notable Seebeck coefficients .
- Quantum Computing Feasibility : Research focusing on ions indicates their potential for stable qubit implementation, with experiments showing promising coherence times suitable for quantum operations .
- Catalytic Efficiency : Investigations into the catalytic properties of ytterbium borides reveal their effectiveness in reducing reaction times significantly compared to traditional catalysts, showcasing their industrial applicability .
作用機序
The mechanism by which ytterbium boride exerts its effects is primarily related to its electronic structure and bonding properties. The compound’s ability to act as a catalyst in chemical reactions is due to the presence of active sites on its surface, which facilitate the adsorption and transformation of reactants. In biological applications, the interaction of this compound with biological tissues is influenced by its surface properties and the release of bioactive ions.
類似化合物との比較
Rare-Earth Hexaborides (e.g., LaB₆, EuB₆)
Property | YbB₆ | LaB₆ | EuB₆ |
---|---|---|---|
Crystal Structure | Cubic (Pm-3m) | Cubic (Pm-3m) | Cubic (Pm-3m) |
Electrical Conductivity | Metallic/semiconducting | Metallic | Semiconducting |
Magnetic Behavior | Paramagnetic (Yb³⁺) | Non-magnetic | Ferromagnetic (Eu²⁺) |
Applications | Thermoelectrics, Kondo studies | Electron emitters | Magnetoresistive devices |
Key Differences :
- YbB₆ and EuB₆ exhibit mixed valence states (Yb²⁺/Yb³⁺, Eu²⁺/Eu³⁺), influencing their electronic properties. LaB₆, in contrast, is a stable metallic conductor used in electron guns .
- YbB₆’s thermoelectric efficiency surpasses LaB₆ due to enhanced phonon scattering from ytterbium’s 4f electrons .
Alkaline Earth Hexaborides (e.g., SrB₆, CaB₆)
Property | YbB₆ | SrB₆ | CaB₆ |
---|---|---|---|
Thermal Conductivity | 2–3 W/m·K | 5–7 W/m·K | 4–6 W/m·K |
Thermoelectric ZT | 0.1–0.2 (300 K) | 0.05–0.1 (300 K) | 0.03–0.08 (300 K) |
Synthesis Method | HPCVD | HPCVD, SPS | Arc melting |
Key Differences :
- YbB₆ exhibits lower thermal conductivity than SrB₆ or CaB₆ due to lattice distortions from smaller ytterbium ions .
- SrB₆ and CaB₆ are often high-pressure densified to enhance mechanical stability, whereas YbB₆ films prioritize phase purity .
Transition Metal Borides (e.g., ZrB₂, TiB₂)
Property | YbB₆ | ZrB₂ | TiB₂ |
---|---|---|---|
Melting Point | ~2,200°C | ~3,250°C | ~3,230°C |
Oxidation Resistance | Moderate (forms Yb₂O₃) | Excellent (forms ZrO₂) | Good (forms TiO₂) |
Applications | Thermoelectrics | Aerospace coatings | Cutting tools |
Key Differences :
- ZrB₂ and TiB₂ are ultra-high-temperature ceramics (UHTCs) with superior oxidation resistance, unlike YbB₆, which degrades above 1,200°C .
- YbB₆ ’s electronic properties are more tunable for energy conversion, while ZrB₂/TiB₂ focus on structural robustness .
Iron Borides (FeB, Fe₂B)
Property | YbB₆ | FeB | Fe₂B |
---|---|---|---|
Hardness (HV) | 15–18 GPa | 20–22 GPa | 18–20 GPa |
Primary Use | Thin-film devices | Wear-resistant coatings | Corrosion barriers |
Synthesis | HPCVD | Pack boriding | Electrochemical deposition |
Key Differences :
生物活性
Ytterbium boride (YbB6) is a compound that has garnered attention in various fields, particularly in biological and medical research. This article provides a detailed overview of its biological activity, including its interactions with biological molecules, potential therapeutic applications, and relevant case studies.
This compound is characterized by its unique properties, which include high thermal stability and electrical conductivity. These attributes make it suitable for various applications, including catalysis and biomedicine. In biological contexts, YbB6 has been explored for its interactions with biomolecules such as DNA and proteins.
The biological activity of this compound primarily arises from its ability to form complexes with biomolecules. These complexes can influence cellular processes through mechanisms such as:
- DNA Cleavage : Yb-complexes exhibit significant DNA cleavage activity, particularly in the presence of oxidative agents like hydrogen peroxide. This property suggests potential applications in cancer therapy where targeted DNA damage can induce apoptosis in malignant cells .
- Protein Binding : Studies have shown that Yb-complexes interact with proteins such as bovine serum albumin (BSA). The binding occurs via hydrophobic interactions and van der Waals forces, with specific binding sites identified through docking studies .
Biological Applications
The biological applications of this compound are diverse, ranging from anticancer therapies to antimicrobial agents.
Anticancer Activity
Recent research indicates that Yb-complexes demonstrate promising cytotoxic effects against various cancer cell lines, including MCF-7 (breast cancer) and A-549 (lung cancer). The cytotoxicity is enhanced when the Yb-complex is encapsulated in nanocarriers, which improve cellular uptake and bioavailability. The half-maximal inhibitory concentration (IC50) values obtained from MTT assays reveal significant anticancer potential .
Cell Line | IC50 (µM) | Treatment |
---|---|---|
MCF-7 | 15.2 | Yb-complex |
A-549 | 12.8 | Yb-complex |
MCF-7 | 8.5 | Starch nano-encapsulated Yb-complex |
A-549 | 7.2 | Lipid nano-encapsulated Yb-complex |
Antimicrobial Activity
This compound exhibits significant antibacterial and antifungal properties. In vitro studies have demonstrated its effectiveness against various Gram-negative bacteria and fungi, with minimum inhibitory concentration (MIC) values indicating robust antimicrobial activity .
Microorganism | MIC (µg/mL) |
---|---|
E. coli | 25 |
S. aureus | 20 |
C. albicans | 15 |
P. aeruginosa | 30 |
Case Studies
- Study on Anticancer Properties : A study investigated the effects of Yb-complexes on MCF-7 and A-549 cell lines using MTT assays to evaluate cell viability post-treatment. Results indicated that the encapsulated forms of the complex significantly enhanced cytotoxicity compared to free complexes .
- Antimicrobial Efficacy Assessment : Another study focused on the antimicrobial properties of Yb-complexes against five different bacterial strains using the zone of inhibition method. The results showed that the complex effectively inhibited bacterial growth at varying concentrations, highlighting its potential as an alternative antimicrobial agent .
- DNA Interaction Studies : Research involving fluorescence spectroscopy and viscosity measurements demonstrated that Yb-complexes bind efficiently to DNA, leading to significant cleavage activity under oxidative conditions. This property is particularly relevant for developing therapeutic agents aimed at inducing DNA damage in cancer cells .
Q & A
Basic Research Questions
Q. What are the primary synthesis methods for high-purity Ytterbium boride (YbB₁₂), and how do process parameters influence crystallographic defects?
- Methodological Answer : Single-crystal YbB₁₂ is typically synthesized via flux growth using aluminum or tin fluxes. Key parameters include cooling rates (e.g., 2–5°C/hour) and annealing durations (48–72 hours) to minimize defects like Yb vacancies . Characterization via X-ray diffraction (XRD) and energy-dispersive spectroscopy (EDS) is critical to verify stoichiometry and lattice symmetry (e.g., cubic structure, Fm-3m space group).
Q. How do temperature-dependent resistivity measurements reveal the Kondo insulating behavior of YbB₁₂?
- Methodological Answer : Below 50 K, resistivity increases logarithmically due to Kondo coherence, while a plateau near 10 K signals hybridization between Yb 4f and boron p orbitals. Four-probe resistivity setups with cryogenic systems (2–300 K) are standard, but surface oxidation must be mitigated via in-situ cleaving or argon glovebox handling .
Q. What spectroscopic techniques are optimal for probing the electronic structure of YbB₁₂?
- Methodological Answer : Angle-resolved photoemission spectroscopy (ARPES) and scanning tunneling microscopy (STM) are pivotal for mapping bulk and surface states. For example, ARPES at synchrotron facilities (e.g., Hiroshima Synchrotron Radiation Center) has identified Dirac-like surface states in YbB₁₂, while STM resolves localized f-electron states .
Advanced Research Questions
Q. How do discrepancies in reported topological surface state (TSS) behaviors of YbB₁₂ arise, and what experimental controls resolve them?
- Methodological Answer : Contradictions in TSS observations (e.g., gap opening vs. gapless spectra) often stem from surface termination or impurity phases. Systematic studies require in-situ surface preparation (e.g., cyclic Ar⁺ sputtering) combined with low-temperature STM (<5 K) to suppress thermal broadening. Cross-validation with density functional theory (DFT+U) calculations is essential to reconcile experimental and theoretical gaps .
Q. What role do spin-orbit coupling (SOC) and strong electron correlations play in the anomalous Hall effect observed in YbB₁₂?
- Methodological Answer : Magnetotransport experiments under high magnetic fields (>10 T) reveal Berry curvature contributions to the Hall conductivity. To isolate SOC effects, comparative studies with isostructural compounds (e.g., SmB₆) are conducted. Advanced DFT+DMFT (dynamical mean-field theory) frameworks model the interplay between SOC and Kondo screening .
Q. How can neutron scattering experiments elucidate magnetic excitations in YbB₁₂, given its non-magnetic ground state?
- Methodological Answer : Inelastic neutron scattering (INS) at facilities like the High Energy Accelerator Research Organization (KEK) probes crystal-field splitting and quadrupolar fluctuations. Polarized neutron setups differentiate between phononic and magnetic signals, critical for resolving hidden magnetic order in Kondo insulators .
Q. What strategies optimize thin-film epitaxy of YbB₁₂ for spintronic device integration?
- Methodological Answer : Molecular beam epitaxy (MBE) on lattice-matched substrates (e.g., MgO) achieves <1% lattice mismatch. In-situ reflection high-energy electron diffraction (RHEED) monitors layer-by-layer growth, while post-annealing in boron-rich atmospheres suppresses interfacial diffusion. Electrical transport in thin films requires microfabrication with non-invasive contacts (e.g., Pt/Ti bilayers) .
Q. Data Analysis and Interpretation
Q. How should researchers address conflicting data on the bulk gap magnitude of YbB₁₂ (10–30 meV range)?
- Methodological Answer : Variations arise from sample quality and measurement techniques. Synchrotron-based ARPES with <5 meV energy resolution provides definitive gap measurements. Statistical averaging across multiple cleaved surfaces and Bayesian error analysis minimize outliers .
Q. What computational frameworks best model the interplay between topology and strong correlations in YbB₁₂?
- Methodological Answer : Hybrid DFT+DMFT approaches, incorporating Wannier function projections for Yb 4f orbitals, capture both topological invariants and Kondo screening. Open-source codes (e.g., Wannier90 and TRIQS) enable reproducibility, with validation against ARPES-derived band structures .
Q. Ethical and Reproducibility Considerations
Q. How can researchers ensure reproducibility in YbB₁₂ studies given sensitivity to synthesis conditions?
- Methodological Answer : Detailed protocols for flux growth (e.g., molar ratios, thermal profiles) must be archived in open-access repositories (e.g., Zenodo). Collaborative round-robin experiments across labs, standardized by institutions like Ibaraki University, establish consensus on intrinsic properties .
Featured Recommendations
Most viewed | ||
---|---|---|
Most popular with customers |
試験管内研究製品の免責事項と情報
BenchChemで提示されるすべての記事および製品情報は、情報提供を目的としています。BenchChemで購入可能な製品は、生体外研究のために特別に設計されています。生体外研究は、ラテン語の "in glass" に由来し、生物体の外で行われる実験を指します。これらの製品は医薬品または薬として分類されておらず、FDAから任何の医療状態、病気、または疾患の予防、治療、または治癒のために承認されていません。これらの製品を人間または動物に体内に導入する形態は、法律により厳格に禁止されています。これらのガイドラインに従うことは、研究と実験において法的および倫理的な基準の遵守を確実にするために重要です。