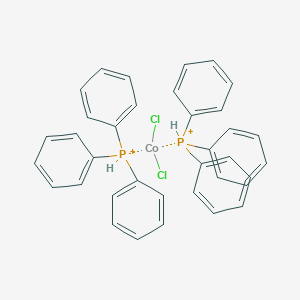
ジクロロビス(トリフェニルホスフィン)コバルト(II)
- 専門家チームからの見積もりを受け取るには、QUICK INQUIRYをクリックしてください。
- 品質商品を競争力のある価格で提供し、研究に集中できます。
概要
説明
Dichlorobis(triphenylphosphine)cobalt(II) is a coordination compound with the chemical formula ([ (C_6H_5)_3P ]_2CoCl_2). It is a cobalt complex where cobalt is bonded to two chloride ions and two triphenylphosphine ligands. This compound is known for its vibrant blue color and is widely used as a catalyst in various chemical reactions .
科学的研究の応用
Dichlorobis(triphenylphosphine)cobalt(II) has a wide range of applications in scientific research, including:
Material Science: It is used in the synthesis of advanced materials, including polymers and nanomaterials.
Medicinal Chemistry:
Biological Studies: It is used in studies involving cobalt’s role in biological systems and its interactions with biomolecules.
作用機序
Target of Action
Dichlorobis(triphenylphosphine)cobalt(II) is primarily used as a catalyst in various chemical reactions . Its primary targets are the reactant molecules in these reactions, where it facilitates the transformation of these molecules into the desired products.
Mode of Action
As a catalyst, Dichlorobis(triphenylphosphine)cobalt(II) interacts with its targets by lowering the activation energy of the reaction, thus accelerating the rate of the reaction . It does this without being consumed in the reaction, meaning it can facilitate multiple reactions consecutively.
Biochemical Pathways
The exact biochemical pathways affected by Dichlorobis(triphenylphosphine)cobalt(II) depend on the specific reactions it is used to catalyze. For instance, it can be used in three-component coupling reactions, epoxidation reactions, hydrovinylation of styrene, cross-coupling reactions, dimerization reactions, and oxidation reactions . The downstream effects of these reactions vary widely and depend on the specific reactants and products involved.
Pharmacokinetics
For instance, it is insoluble in water , which can affect its use in certain reactions.
Result of Action
The result of Dichlorobis(triphenylphosphine)cobalt(II)'s action is the facilitation of chemical reactions, leading to the formation of the desired products more quickly and efficiently than would be possible without the catalyst .
Action Environment
The action of Dichlorobis(triphenylphosphine)cobalt(II) can be influenced by various environmental factors. For example, the presence of water or certain other solvents can impact its effectiveness due to its insolubility in water . Additionally, factors such as temperature and pressure can also affect the rate of the catalyzed reactions.
準備方法
Synthetic Routes and Reaction Conditions
Dichlorobis(triphenylphosphine)cobalt(II) can be synthesized through the reaction of cobalt(II) chloride with triphenylphosphine in an inert atmosphere. The reaction typically involves dissolving cobalt(II) chloride in a suitable solvent such as ethanol or methanol, followed by the addition of triphenylphosphine. The mixture is then stirred under an inert atmosphere (e.g., nitrogen or argon) to prevent oxidation .
Industrial Production Methods
While specific industrial production methods for dichlorobis(triphenylphosphine)cobalt(II) are not extensively documented, the synthesis generally follows similar principles as laboratory preparation, with considerations for scaling up the process. This includes maintaining an inert atmosphere and using larger quantities of reagents and solvents .
化学反応の分析
Types of Reactions
Dichlorobis(triphenylphosphine)cobalt(II) undergoes various types of reactions, including:
Oxidation: The compound can be oxidized to higher oxidation states of cobalt.
Reduction: It can be reduced to lower oxidation states or even to metallic cobalt.
Substitution: The chloride ligands can be substituted with other ligands such as bromides or iodides.
Common Reagents and Conditions
Common reagents used in reactions with dichlorobis(triphenylphosphine)cobalt(II) include:
Oxidizing agents: Such as hydrogen peroxide or oxygen.
Reducing agents: Such as sodium borohydride or lithium aluminum hydride.
Substituting agents: Such as halide salts (e.g., sodium bromide, sodium iodide).
Major Products Formed
The major products formed from these reactions depend on the specific reagents and conditions used. For example:
Oxidation: Can lead to the formation of cobalt(III) complexes.
Reduction: Can result in the formation of cobalt(I) complexes or metallic cobalt.
Substitution: Can produce dichlorobis(triphenylphosphine)cobalt(II) derivatives with different halides.
類似化合物との比較
Similar Compounds
Bis(triphenylphosphine)nickel(II) dichloride: Similar in structure but contains nickel instead of cobalt.
Chlorotris(triphenylphosphine)cobalt(I): Contains one additional triphenylphosphine ligand and cobalt in a different oxidation state.
Bis(triphenylphosphine)palladium(II) dichloride: Contains palladium instead of cobalt and is used in similar catalytic applications.
Uniqueness
Dichlorobis(triphenylphosphine)cobalt(II) is unique due to its specific electronic properties and reactivity profile, which are influenced by the cobalt center and the triphenylphosphine ligands. Its ability to participate in a wide range of catalytic reactions makes it a valuable compound in both academic and industrial research .
特性
CAS番号 |
14126-40-0 |
---|---|
分子式 |
C36H30Cl2CoP2 |
分子量 |
654.4 g/mol |
IUPAC名 |
cobalt(2+);triphenylphosphane;dichloride |
InChI |
InChI=1S/2C18H15P.2ClH.Co/c2*1-4-10-16(11-5-1)19(17-12-6-2-7-13-17)18-14-8-3-9-15-18;;;/h2*1-15H;2*1H;/q;;;;+2/p-2 |
InChIキー |
OPRPFIIIFJLFCE-UHFFFAOYSA-L |
SMILES |
C1=CC=C(C=C1)P(C2=CC=CC=C2)C3=CC=CC=C3.C1=CC=C(C=C1)P(C2=CC=CC=C2)C3=CC=CC=C3.Cl[Co]Cl |
正規SMILES |
C1=CC=C(C=C1)P(C2=CC=CC=C2)C3=CC=CC=C3.C1=CC=C(C=C1)P(C2=CC=CC=C2)C3=CC=CC=C3.[Cl-].[Cl-].[Co+2] |
製品の起源 |
United States |
Retrosynthesis Analysis
AI-Powered Synthesis Planning: Our tool employs the Template_relevance Pistachio, Template_relevance Bkms_metabolic, Template_relevance Pistachio_ringbreaker, Template_relevance Reaxys, Template_relevance Reaxys_biocatalysis model, leveraging a vast database of chemical reactions to predict feasible synthetic routes.
One-Step Synthesis Focus: Specifically designed for one-step synthesis, it provides concise and direct routes for your target compounds, streamlining the synthesis process.
Accurate Predictions: Utilizing the extensive PISTACHIO, BKMS_METABOLIC, PISTACHIO_RINGBREAKER, REAXYS, REAXYS_BIOCATALYSIS database, our tool offers high-accuracy predictions, reflecting the latest in chemical research and data.
Strategy Settings
Precursor scoring | Relevance Heuristic |
---|---|
Min. plausibility | 0.01 |
Model | Template_relevance |
Template Set | Pistachio/Bkms_metabolic/Pistachio_ringbreaker/Reaxys/Reaxys_biocatalysis |
Top-N result to add to graph | 6 |
Feasible Synthetic Routes
Q1: What insights do the studies offer regarding the structural characterization of Dichlorobis(triphenylphosphine)cobalt(II)?
A1: While the exact molecular formula and weight aren't explicitly provided in the abstracts, one study focuses on deriving dipolar nuclear magnetic resonance shifts directly from single-crystal magnetic susceptibilities of Dichlorobis(triphenylphosphine)cobalt(II) []. This suggests the compound's paramagnetic nature and provides a method to analyze its magnetic anisotropy, a crucial aspect influencing its interactions and reactivity. Another study investigates the polarized spectrum of Dichlorobis(triphenylphosphine)cobalt(II) [], offering valuable information about its electronic structure and how it interacts with light. This spectroscopic data is vital for understanding the compound's behavior in various chemical environments.
Q2: How does Dichlorobis(triphenylphosphine)cobalt(II) interact with other molecules, specifically crown ethers?
A2: A research paper directly investigates the "Complexations of dichlorobis(triphenylphosphine)cobalt(II) with crown ethers" []. This study likely explores the coordination chemistry of the compound, examining how the central cobalt atom interacts with the oxygen atoms in crown ethers to form complexes. Understanding these interactions is critical for predicting the compound's behavior in systems containing crown ethers and could have implications for designing specific reactions or separation processes.
Q3: Are there any computational chemistry approaches mentioned in the research that could be applied to Dichlorobis(triphenylphosphine)cobalt(II)?
A3: Although no studies explicitly mention computational modeling for this specific compound, the use of single-crystal magnetic susceptibilities to derive NMR shifts in one study [] suggests that computational methods could be implemented. Techniques like Density Functional Theory (DFT) calculations could be employed to model the electronic structure and predict spectroscopic properties of Dichlorobis(triphenylphosphine)cobalt(II), further refining our understanding of its behavior.
試験管内研究製品の免責事項と情報
BenchChemで提示されるすべての記事および製品情報は、情報提供を目的としています。BenchChemで購入可能な製品は、生体外研究のために特別に設計されています。生体外研究は、ラテン語の "in glass" に由来し、生物体の外で行われる実験を指します。これらの製品は医薬品または薬として分類されておらず、FDAから任何の医療状態、病気、または疾患の予防、治療、または治癒のために承認されていません。これらの製品を人間または動物に体内に導入する形態は、法律により厳格に禁止されています。これらのガイドラインに従うことは、研究と実験において法的および倫理的な基準の遵守を確実にするために重要です。