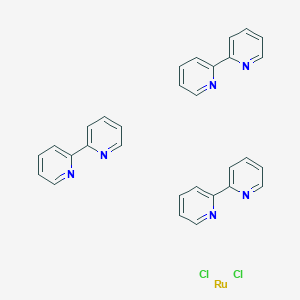
Dichlororuthenium;2-pyridin-2-ylpyridine
説明
Dichlororuthenium;2-pyridin-2-ylpyridine, formally known as cis-bis(2,2'-bipyridine)dichlororuthenium(II) hydrate, is a ruthenium-based coordination complex with the formula C₂₀H₁₆Cl₂N₄Ru·xH₂O . This compound features a central ruthenium(II) ion coordinated by two bidentate 2,2'-bipyridine (bpy) ligands and two chloride ions in a cis configuration, with additional water molecules in the hydrate form . Its molecular weight is 484.35 g/mol, and it appears as a black-to-green crystalline powder with 97% purity in commercial forms .
The complex is water-soluble, making it suitable for applications in aqueous chemistry and biomedicine . It serves as a precursor for synthesizing luminescent ruthenium polypyridyl complexes, which are investigated for their roles in radiosensitization for cancer therapy, particularly in enhancing radiation-induced DNA damage in pancreatic cancer cells . Its stability and redox activity are attributed to the strong π-accepting and σ-donating properties of the bipyridine ligands, which stabilize the Ru(II) center and enable tunable electronic transitions .
生物活性
Dichlororuthenium;2-pyridin-2-ylpyridine is a ruthenium complex that has garnered attention for its diverse biological activities, particularly in the fields of cancer therapy and antibacterial applications. This article explores the compound's mechanisms of action, efficacy in various biological contexts, and potential applications based on recent research findings.
Chemical Structure and Properties
The compound is characterized by its coordination with two pyridine ligands and two chloride ions, forming a stable ruthenium complex. Its molecular formula is , with a molecular weight of approximately 320.14 g/mol. The structure allows for significant interactions with biological macromolecules, which underpins its biological activity.
1. Anticancer Properties
Research indicates that dichlororuthenium complexes can induce apoptosis in cancer cells through several mechanisms:
- Oxidative Stress : The compound generates reactive oxygen species (ROS) that can damage cellular components, leading to cell death.
- DNA Interaction : Studies have shown that dichlororuthenium can bind to DNA, interfering with replication and transcription processes. This interaction may lead to the formation of DNA adducts, which can trigger cellular repair mechanisms or apoptosis if the damage is irreparable .
2. Antibacterial Activity
Dichlororuthenium complexes have demonstrated antibacterial effects against both Gram-positive and Gram-negative bacteria. For instance:
- Minimum Inhibitory Concentration (MIC) : Research has reported MIC values of 35 µg/mL against Staphylococcus aureus and 65 µg/mL against Escherichia coli, indicating significant antibacterial potency .
- Mechanism of Action : The antibacterial effects are attributed to the complex's ability to disrupt bacterial cell membranes and interfere with metabolic processes by altering pH levels within bacterial cells .
Case Study 1: Anticancer Efficacy
A study investigated the cytotoxic effects of dichlororuthenium on various cancer cell lines, including breast and prostate cancer cells. The results showed a dose-dependent increase in cell death, with IC50 values significantly lower than those of traditional chemotherapeutics. The study concluded that the compound's ability to induce oxidative stress was a primary mechanism for its anticancer activity .
Case Study 2: Antibacterial Activity
In another investigation, the antibacterial properties of dichlororuthenium were tested against multiple bacterial strains using standard broth dilution methods. The compound exhibited bacteriostatic effects at lower concentrations and bactericidal effects at higher concentrations. The study highlighted its potential as a novel antibacterial agent in treating infections caused by resistant bacterial strains .
Comparative Analysis of Related Compounds
To understand the unique properties of this compound, it is beneficial to compare it with similar ruthenium complexes:
Compound Name | Molecular Formula | Anticancer Activity | Antibacterial Activity |
---|---|---|---|
This compound | C10H8Cl2N2Ru | High | Moderate |
cis-Dichlorobis(1,10-phenanthroline)ruthenium(II) | C22H16Cl2N4Ru | Moderate | Low |
Tris(2,2'-bipyridyl)dichlororuthenium(II) hexahydrate | C30H24Cl2N6Ru·6H2O | High | High |
This table illustrates that while this compound exhibits strong anticancer properties, its antibacterial activity is moderate compared to other ruthenium complexes.
科学的研究の応用
Catalytic Applications
Catalysis in Organic Reactions
- Cross-Coupling Reactions : The compound serves as an effective catalyst in cross-coupling reactions, facilitating the formation of carbon-carbon bonds. This is particularly important in organic synthesis for pharmaceuticals and agrochemicals .
- Photoredox Catalysis : It is utilized in photoredox catalysis, where light energy is harnessed to drive chemical reactions. This includes C-H activation and other organic transformations .
Table 1: Catalytic Applications of Dichlororuthenium;2-pyridin-2-ylpyridine
Application Type | Specific Use | Reference |
---|---|---|
Cross-Coupling | Formation of carbon-carbon bonds | |
Photoredox Catalysis | C-H activation | |
Water Splitting | Photochemical water splitting studies |
Photochemical Applications
Photochemistry and Light Emission
- Solar Energy Conversion : The compound exhibits metal-to-ligand charge transfer upon light absorption, making it a candidate for solar energy applications .
- Light-Emitting Devices : Its luminescent properties are exploited in the development of light-emitting diodes (LEDs) and other optoelectronic devices .
Biological Applications
Bioimaging and Sensing
- Oxygen Detection : The compound is employed as a luminescent probe for detecting oxygen levels in biological systems. This has implications for monitoring cellular environments and metabolic processes .
- Anticancer Research : Studies have shown that dichlororuthenium complexes can induce apoptosis in cancer cells, suggesting potential as anticancer agents. Their mechanism involves oxidative stress pathways leading to DNA damage .
Table 2: Biological Applications of this compound
Application Type | Specific Use | Reference |
---|---|---|
Bioimaging | Luminescent probe for oxygen detection | |
Anticancer Research | Induction of apoptosis |
Industrial Applications
Electrochemical Sensors
- Smart Biosensors : The compound is used in the development of electrochemiluminescence sensors for point-of-care testing and environmental monitoring .
- Photovoltaic Cells : It plays a role in the development of photoelectrical components for photovoltaic applications, enhancing energy conversion efficiency .
Case Studies
Case Study 1: Photoredox Catalysis in Organic Synthesis
Research demonstrated the effectiveness of dichlororuthenium complexes in facilitating photoredox reactions that lead to the synthesis of complex organic molecules. The study highlighted its ability to promote C-C bond formation under mild conditions, showcasing its utility in pharmaceutical chemistry.
Case Study 2: Anticancer Activity Assessment
A series of experiments were conducted to evaluate the cytotoxic effects of dichlororuthenium on various cancer cell lines. Results indicated significant induction of apoptosis through oxidative stress mechanisms, paving the way for further investigations into its therapeutic potential.
Q & A
Basic Research Questions
Q. What are the standard characterization techniques for confirming the structure of dichlororuthenium;2-pyridin-2-ylpyridine complexes?
Q. How to optimize the synthesis of this compound for high purity?
Methodological Answer: Synthesis optimization involves:
- Precursor Selection : Use anhydrous RuCl₃ to minimize hydrolysis side products.
- Solvent Choice : Dichloromethane or ethanol for ligand exchange reactions, ensuring solubility of intermediates .
- Stoichiometric Control : Maintain a 1:2 molar ratio of RuCl₃ to 2,2'-bipyridine to prevent ligand excess.
- Purification : Recrystallization from hot water or ethanol removes unreacted ligands. Purity >96% is achievable via repeated washing .
- Hydration Management : Dihydrate forms (e.g., RuCl₂(bpy)₂·2H₂O) require controlled drying to avoid variable water content .
Critical Step : Conduct reactions under inert atmosphere (N₂/Ar) to prevent Ru(II) oxidation to Ru(III) .
Advanced Research Questions
Q. What methodologies resolve contradictions in reported catalytic activities of this compound across solvent systems?
Methodological Answer: Discrepancies in catalytic efficiency (e.g., in water vs. organic solvents) arise from solvent polarity, counterion effects, and hydration state. Address these via:
- Systematic Solvent Screening : Test catalytic performance in aprotic (DMF, acetonitrile) vs. protic (water, ethanol) solvents. Solubility data (e.g., water-soluble form ) guides solvent selection.
- Counterion Studies : Replace chloride with BF₄⁻ or PF₆⁻ to assess ion-pairing effects on redox potentials.
- In situ Spectroelectrochemistry : Monitor Ru(II/III) transitions under catalytic conditions to correlate activity with oxidation states .
- Computational Modeling : Use DFT to predict solvent interactions and transition-state energetics (e.g., ICReDD’s reaction path search methods ).
Example Workflow :
- Vary solvent polarity and measure turnover frequency (TOF).
- Cross-validate with cyclic voltammetry to link catalytic rates to Ru redox behavior.
Q. How can computational modeling predict the photophysical behavior of this compound in novel applications?
Methodological Answer: Photophysical properties (e.g., MLCT transitions) are key for light-driven applications. Integrate:
- TD-DFT Calculations : Simulate electronic transitions and compare with experimental UV-Vis spectra. Adjust basis sets (e.g., LANL2DZ for Ru) for accuracy .
- Ligand Substitution Modeling : Predict how modifying bipyridine substituents alters absorption/emission maxima.
- Solvent Embedding : Include solvent effects (e.g., COSMO model) to refine excited-state lifetimes .
- Experimental Validation : Use transient absorption spectroscopy to verify computed excited-state dynamics.
Case Study : For biomedical applications (e.g., photodynamic therapy), model singlet oxygen quantum yields via intersystem crossing rates .
Q. Safety and Handling Considerations
Q. What safety protocols are critical when handling this compound in air-sensitive experiments?
Methodological Answer:
- Personal Protective Equipment (PPE) : Wear nitrile gloves, lab coat, and safety goggles. Use face shields during sonication .
- Ventilation : Conduct reactions in a fume hood to avoid inhalation of fine powders (GHS H335) .
- Spill Management : Neutralize aqueous solutions with NaHCO₃ before disposal.
- Storage : Keep in airtight containers under N₂ to prevent hydration/decomposition .
GHS Compliance :
Hazard Code | Risk Statement | Precautionary Measure |
---|---|---|
H315 | Skin irritation | Wear protective gloves |
H319 | Serious eye irritation | Use eye protection |
H335 | Respiratory irritation | Avoid dust inhalation |
Q. Data Contradiction Analysis
Q. How to address conflicting reports on the hydration state’s impact on catalytic performance?
Methodological Answer:
- Controlled Hydration Studies : Synthesize anhydrous and dihydrate forms (e.g., RuCl₂(bpy)₂ vs. RuCl₂(bpy)₂·2H₂O) and compare catalytic rates in identical conditions .
- Thermogravimetric Analysis (TGA) : Quantify water loss during catalysis to correlate activity with hydration state.
- Isotopic Labeling : Use D₂O to track water’s role in proton-coupled electron transfer (PCET) mechanisms .
Resolution Example : If dihydrate forms show lower activity in organic solvents, attribute this to water-ligand competition for Ru coordination sites.
類似化合物との比較
Structural and Functional Analogues
cis-Bis(2,2'-bipyridine)dichloroplatinum(II)
- Formula : C₁₀H₈Cl₂N₂Pt .
- Structure : Platinum(II) center with two bpy ligands and two chlorides in cis configuration.
- Key Differences: Metal Center: Platinum(II) vs. Ruthenium(II). Pt(II) complexes are typically square planar, whereas Ru(II) adopts an octahedral geometry. Applications: Platinum analogues (e.g., cisplatin) are widely used in chemotherapy but are nephrotoxic. The Ru(II) complex exhibits lower systemic toxicity and unique photophysical properties for radiosensitization . Solubility: Limited water solubility for Pt complexes compared to the Ru analogue .
Tris(2,2'-bipyridine)ruthenium(II) Chloride Hexahydrate
- Formula : [Ru(bpy)₃]Cl₂·6H₂O .
- Structure : Ru(II) center with three bpy ligands.
- Key Differences :
- Ligand Count : Three bpy ligands vs. two in the dichlororuthenium complex.
- Charge : [Ru(bpy)₃]²⁺ carries a +2 charge, while [Ru(bpy)₂Cl₂] is neutral.
- Applications : Used in electrochemiluminescence (ECL) sensors and light-harvesting systems due to intense luminescence, unlike the dichloro variant’s focus on radiosensitization .
Tetrakis(triphenylphosphine)dichlororuthenium(II)
- Formula : RuCl₂(PPh₃)₄ .
- Structure: Ru(II) with four monodentate triphenylphosphine (PPh₃) ligands and two chlorides.
- Key Differences: Ligand Type: PPh₃ (strong σ-donor, weak π-acceptor) vs. bpy (strong π-acceptor). Reactivity: PPh₃ complexes are more reactive in catalysis (e.g., hydrogenation), while bpy complexes prioritize stability and photoredox activity .
Key Research Findings
Biomedical Efficacy : The Ru(II) bipyridine complex enhances radiation-induced DNA damage in pancreatic cancer cells at 10 µM concentration , outperforming platinum analogues in tumor growth inhibition .
Luminescence : While [Ru(bpy)₃]²⁺ emits at 620 nm (ECL applications), the dichloro variant shows quenched luminescence due to chloride ligand dissociation .
Catalytic Activity : RuCl₂(PPh₃)₄ demonstrates 90% yield in hydrogenation reactions, whereas the bipyridine complex is inert under similar conditions .
特性
IUPAC Name |
dichlororuthenium;2-pyridin-2-ylpyridine | |
---|---|---|
Source | PubChem | |
URL | https://pubchem.ncbi.nlm.nih.gov | |
Description | Data deposited in or computed by PubChem | |
InChI |
InChI=1S/3C10H8N2.2ClH.Ru/c3*1-3-7-11-9(5-1)10-6-2-4-8-12-10;;;/h3*1-8H;2*1H;/q;;;;;+2/p-2 | |
Source | PubChem | |
URL | https://pubchem.ncbi.nlm.nih.gov | |
Description | Data deposited in or computed by PubChem | |
InChI Key |
SJFYGUKHUNLZTK-UHFFFAOYSA-L | |
Source | PubChem | |
URL | https://pubchem.ncbi.nlm.nih.gov | |
Description | Data deposited in or computed by PubChem | |
Canonical SMILES |
C1=CC=NC(=C1)C2=CC=CC=N2.C1=CC=NC(=C1)C2=CC=CC=N2.C1=CC=NC(=C1)C2=CC=CC=N2.Cl[Ru]Cl | |
Source | PubChem | |
URL | https://pubchem.ncbi.nlm.nih.gov | |
Description | Data deposited in or computed by PubChem | |
Molecular Formula |
C30H24Cl2N6Ru | |
Source | PubChem | |
URL | https://pubchem.ncbi.nlm.nih.gov | |
Description | Data deposited in or computed by PubChem | |
Molecular Weight |
640.5 g/mol | |
Source | PubChem | |
URL | https://pubchem.ncbi.nlm.nih.gov | |
Description | Data deposited in or computed by PubChem | |
Physical Description |
Hydrate: Orange to red crystalline powder with a mild odor; [GFS Chemicals MSDS] | |
Record name | Tris(2,2'-bipyridine)ruthenium dichloride | |
Source | Haz-Map, Information on Hazardous Chemicals and Occupational Diseases | |
URL | https://haz-map.com/Agents/17161 | |
Description | Haz-Map® is an occupational health database designed for health and safety professionals and for consumers seeking information about the adverse effects of workplace exposures to chemical and biological agents. | |
Explanation | Copyright (c) 2022 Haz-Map(R). All rights reserved. Unless otherwise indicated, all materials from Haz-Map are copyrighted by Haz-Map(R). No part of these materials, either text or image may be used for any purpose other than for personal use. Therefore, reproduction, modification, storage in a retrieval system or retransmission, in any form or by any means, electronic, mechanical or otherwise, for reasons other than personal use, is strictly prohibited without prior written permission. | |
CAS No. |
14323-06-9 | |
Record name | Ruthenium(2+), tris(2,2'-bipyridine-.kappa.N1,.kappa.N1')-, chloride (1:2), (OC-6-11)- | |
Source | EPA Chemicals under the TSCA | |
URL | https://www.epa.gov/chemicals-under-tsca | |
Description | EPA Chemicals under the Toxic Substances Control Act (TSCA) collection contains information on chemicals and their regulations under TSCA, including non-confidential content from the TSCA Chemical Substance Inventory and Chemical Data Reporting. | |
Record name | Tris[2,2'-bipyridine]ruthenium dichloride | |
Source | European Chemicals Agency (ECHA) | |
URL | https://echa.europa.eu/substance-information/-/substanceinfo/100.034.772 | |
Description | The European Chemicals Agency (ECHA) is an agency of the European Union which is the driving force among regulatory authorities in implementing the EU's groundbreaking chemicals legislation for the benefit of human health and the environment as well as for innovation and competitiveness. | |
Explanation | Use of the information, documents and data from the ECHA website is subject to the terms and conditions of this Legal Notice, and subject to other binding limitations provided for under applicable law, the information, documents and data made available on the ECHA website may be reproduced, distributed and/or used, totally or in part, for non-commercial purposes provided that ECHA is acknowledged as the source: "Source: European Chemicals Agency, http://echa.europa.eu/". Such acknowledgement must be included in each copy of the material. ECHA permits and encourages organisations and individuals to create links to the ECHA website under the following cumulative conditions: Links can only be made to webpages that provide a link to the Legal Notice page. | |
試験管内研究製品の免責事項と情報
BenchChemで提示されるすべての記事および製品情報は、情報提供を目的としています。BenchChemで購入可能な製品は、生体外研究のために特別に設計されています。生体外研究は、ラテン語の "in glass" に由来し、生物体の外で行われる実験を指します。これらの製品は医薬品または薬として分類されておらず、FDAから任何の医療状態、病気、または疾患の予防、治療、または治癒のために承認されていません。これらの製品を人間または動物に体内に導入する形態は、法律により厳格に禁止されています。これらのガイドラインに従うことは、研究と実験において法的および倫理的な基準の遵守を確実にするために重要です。