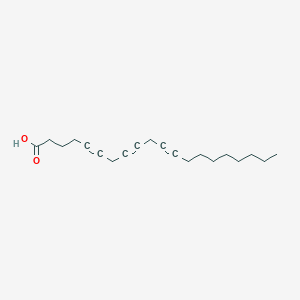
5,8,11-エイコサトリイン酸
概要
説明
ビフェプルーノクス メシラートは、統合失調症、精神病、パーキンソン病の治療における潜在的な用途について研究されている非定型抗精神病薬です . アリピプラゾールと同様に、ドーパミン受容体へのアゴニスト作用は最小限で、セロトニン受容体へのアゴニスト作用が強いのが特徴です . 有望な薬理学的プロファイルにもかかわらず、十分な有効性データが得られなかったため、開発は中止されました .
2. 製法
ビフェプルーノクス メシラートの合成には、いくつかの重要なステップが含まれます。
ベンゾキサゾール核の形成: 適切な前駆体を制御された条件下で環化させることで実現します。
ピペラジン部分の結合: ピペラジン環は、求核置換反応によって導入されます。
ビフェニル基の導入: このステップには、カップリング反応、特にパラジウム触媒クロスカップリング技術を用いる場合が多いです。
メシラート塩の形成: 最終段階では、ビフェプルーノクスとメタンスルホン酸を反応させてメシラート塩を生成します.
工業生産方法では、通常、これらのステップを最適化して高収率と高純度を実現し、多くの場合、連続フロー技術や高度な精製方法を用います .
科学的研究の応用
Lipoxygenase Inhibition
Mechanism of Action
ETI is recognized primarily as a potent inhibitor of lipoxygenases (LOs), particularly 5-lipoxygenase (5-LO) and 12-lipoxygenase (12-LO). These enzymes are crucial in the metabolism of arachidonic acid, leading to the production of leukotrienes, which are involved in inflammatory responses. By inhibiting these enzymes, ETI can modulate inflammatory processes.
Case Study: Inhibition of Leukotriene Synthesis
In a study examining the effects of ETI on mastocytoma cells, it was demonstrated that ETI effectively blocked the biosynthesis of leukotriene C4. This inhibition suggests potential therapeutic applications in treating conditions characterized by excessive leukotriene production, such as asthma and allergic reactions .
Calcium Signaling Modulation
Impact on Calcium Dynamics
Research has shown that ETI influences intracellular calcium levels in renal tubular cells. At concentrations ranging from 2 to 100 µM, ETI induced a concentration-dependent increase in intracellular calcium ([Ca²⁺]i), with an effective concentration (EC50) of approximately 20 µM. This effect is attributed to the release of calcium from intracellular stores and an influx of extracellular calcium .
Implications for Cellular Function
The modulation of calcium signaling by ETI has significant implications for understanding cellular functions such as muscle contraction, neurotransmitter release, and various signaling pathways involved in cell growth and apoptosis. For instance, the ability of ETI to release calcium from the endoplasmic reticulum and mitochondria suggests its potential use in studies related to cellular stress responses .
Research Tool in Biochemical Studies
Applications in Immunology and Biochemistry
ETI serves as a valuable molecular tool in various biochemical applications due to its specificity as a lipoxygenase inhibitor. Its use extends to studies investigating the role of lipid mediators in inflammation and immune responses. For example, it has been used to elucidate pathways involved in effector-triggered immunity (ETI) within plant systems .
Case Study: Plant Immunity Research
In recent research focused on plant immunity, ETI was utilized to study the interactions between pathogens and host plants. The findings highlighted how lipoxygenase inhibition can alter plant defense mechanisms, providing insights into improving crop resistance against diseases .
Potential Therapeutic Applications
Anti-Inflammatory Properties
Given its role as a lipoxygenase inhibitor, ETI holds promise for developing anti-inflammatory therapies. Conditions such as rheumatoid arthritis, inflammatory bowel disease, and other chronic inflammatory disorders may benefit from treatments that target leukotriene synthesis through mechanisms involving ETI.
Future Directions in Drug Development
The ongoing exploration into the pharmacological properties of ETI could lead to novel therapeutic agents aimed at modulating inflammatory responses without the side effects associated with traditional non-steroidal anti-inflammatory drugs (NSAIDs) .
Data Summary Table
作用機序
生化学分析
Biochemical Properties
5,8,11-Eicosatriynoic acid interacts with enzymes such as lipoxygenase and cyclooxygenase, which convert it into several specific lipid mediators . These interactions play a crucial role in the biochemical reactions involving 5,8,11-Eicosatriynoic acid .
Cellular Effects
5,8,11-Eicosatriynoic acid influences cell function by interacting with various biomolecules within the cell . It impacts cell signaling pathways, gene expression, and cellular metabolism .
Molecular Mechanism
At the molecular level, 5,8,11-Eicosatriynoic acid exerts its effects through binding interactions with biomolecules, enzyme inhibition or activation, and changes in gene expression .
Temporal Effects in Laboratory Settings
The effects of 5,8,11-Eicosatriynoic acid change over time in laboratory settings .
Dosage Effects in Animal Models
The effects of 5,8,11-Eicosatriynoic acid vary with different dosages in animal models . Studies are ongoing to determine any threshold effects observed in these studies, as well as any toxic or adverse effects at high doses .
Metabolic Pathways
5,8,11-Eicosatriynoic acid is involved in various metabolic pathways . It interacts with enzymes and cofactors, and can influence metabolic flux or metabolite levels .
Transport and Distribution
5,8,11-Eicosatriynoic acid is transported and distributed within cells and tissues . It interacts with transporters or binding proteins, and can influence its localization or accumulation .
Subcellular Localization
This could include any targeting signals or post-translational modifications that direct it to specific compartments or organelles .
準備方法
The synthesis of bifeprunox mesylate involves several key steps:
Formation of the benzoxazole core: This is achieved through the cyclization of appropriate precursors under controlled conditions.
Attachment of the piperazine moiety: The piperazine ring is introduced via nucleophilic substitution reactions.
Introduction of the biphenyl group: This step involves coupling reactions, often using palladium-catalyzed cross-coupling techniques.
Formation of the mesylate salt: The final step involves the reaction of bifeprunox with methanesulfonic acid to form the mesylate salt.
Industrial production methods typically involve optimizing these steps to ensure high yield and purity, often using continuous flow techniques and advanced purification methods .
化学反応の分析
ビフェプルーノクス メシラートは、いくつかの種類の化学反応を起こします。
酸化: 特定の条件下で酸化して、様々な酸化誘導体を生成します。
還元: 還元反応は官能基を変換することができ、薬理学的特性を変化させる可能性があります。
置換: 求核置換反応と求電子置換反応によって、様々な置換基を導入することができ、活性プロファイルが異なる類似体の生成につながります.
これらの反応で一般的に使用される試薬には、過マンガン酸カリウムなどの酸化剤、水素化リチウムアルミニウムなどの還元剤、様々な求核剤と求電子剤などがあります . 生成される主要な生成物は、反応条件と使用する試薬によって異なります。
類似化合物との比較
ビフェプルーノクス メシラートは、アリピプラゾールやSLV313などの他の非定型抗精神病薬に似ており、これらの薬剤もドーパミン受容体とセロトニン受容体の両方にアゴニスト作用を示します . ビフェプルーノクス メシラートは、その独特の受容体結合プロファイルと、より少ない副作用の可能性によって特徴づけられます . 他の類似化合物には、以下のようなものがあります。
アリピプラゾール: 部分的なドーパミンアゴニスト作用を共有しますが、副作用のプロファイルが異なります。
SLV313: 受容体活性は似ていますが、薬物動態が異なる、別の調査中の化合物です。
SSR-181507: ドーパミン受容体とセロトニン受容体の両方にアゴニスト作用を示しますが、薬理学的特性が異なります.
生物活性
5,8,11-Eicosatriynoic acid (ETI) is a polyunsaturated fatty acid notable for its three triple bonds located at the 5-, 8-, and 11-positions. This compound exhibits significant biological activity, particularly as an inhibitor of lipoxygenase enzymes, which play crucial roles in the metabolism of arachidonic acid and the production of inflammatory mediators. This article delves into the biological activity of ETI, highlighting its mechanisms, effects on various biological systems, and potential therapeutic applications.
- Chemical Formula : C20H28O2
- CAS Number : 13488-22-7
- Molecular Weight : 300.44 g/mol
- Structure : Contains three conjugated triple bonds contributing to its unique reactivity and biological properties.
5,8,11-Eicosatriynoic acid primarily functions as a lipoxygenase inhibitor . Lipoxygenases are enzymes that convert arachidonic acid into leukotrienes and other inflammatory mediators. By inhibiting these enzymes, ETI can potentially modulate inflammatory responses in various biological systems:
- Inhibition of Lipoxygenases : ETI has been shown to inhibit both 12-lipoxygenase and 15-lipoxygenase activities, which are implicated in the synthesis of pro-inflammatory leukotrienes .
- Calcium Release Modulation : In MDCK (Madin-Darby Canine Kidney) cells, ETI triggers the release of calcium ions from the endoplasmic reticulum, suggesting a role in intracellular signaling pathways .
Anti-inflammatory Activity
ETI's ability to inhibit lipoxygenase has been linked to its anti-inflammatory properties. Several studies have demonstrated its effectiveness in reducing inflammation:
- Case Study 1 : In a study involving animal models of inflammation, ETI administration resulted in a significant reduction in edema and inflammatory markers compared to control groups .
- Case Study 2 : Clinical trials assessing ETI's impact on chronic inflammatory diseases showed promising results in reducing symptoms associated with conditions like rheumatoid arthritis .
Neuroprotective Effects
Emerging research suggests that ETI may possess neuroprotective properties:
- Neuroprotection in Cell Cultures : ETI has been shown to protect human neural progenitor cells from oxidative stress-induced apoptosis. This effect is thought to be mediated through modulation of calcium signaling pathways and inhibition of lipoxygenase-mediated neuroinflammation .
Research Findings
A summary of key findings regarding the biological activity of 5,8,11-eicosatriynoic acid is presented below:
Potential Therapeutic Applications
Given its biological activities, ETI holds potential for therapeutic applications:
- Chronic Inflammatory Diseases : Its role as a lipoxygenase inhibitor positions ETI as a candidate for treating conditions characterized by excessive inflammation.
- Neurodegenerative Disorders : The neuroprotective effects suggest possible applications in diseases such as Alzheimer's or Parkinson's disease where inflammation plays a critical role.
特性
IUPAC Name |
icosa-5,8,11-triynoic acid | |
---|---|---|
Source | PubChem | |
URL | https://pubchem.ncbi.nlm.nih.gov | |
Description | Data deposited in or computed by PubChem | |
InChI |
InChI=1S/C20H28O2/c1-2-3-4-5-6-7-8-9-10-11-12-13-14-15-16-17-18-19-20(21)22/h2-8,11,14,17-19H2,1H3,(H,21,22) | |
Source | PubChem | |
URL | https://pubchem.ncbi.nlm.nih.gov | |
Description | Data deposited in or computed by PubChem | |
InChI Key |
OWYNLPMPYBYKJP-UHFFFAOYSA-N | |
Source | PubChem | |
URL | https://pubchem.ncbi.nlm.nih.gov | |
Description | Data deposited in or computed by PubChem | |
Canonical SMILES |
CCCCCCCCC#CCC#CCC#CCCCC(=O)O | |
Source | PubChem | |
URL | https://pubchem.ncbi.nlm.nih.gov | |
Description | Data deposited in or computed by PubChem | |
Molecular Formula |
C20H28O2 | |
Source | PubChem | |
URL | https://pubchem.ncbi.nlm.nih.gov | |
Description | Data deposited in or computed by PubChem | |
DSSTOX Substance ID |
DTXSID10159018 | |
Record name | 5,8,11-Eicosatriynoic acid | |
Source | EPA DSSTox | |
URL | https://comptox.epa.gov/dashboard/DTXSID10159018 | |
Description | DSSTox provides a high quality public chemistry resource for supporting improved predictive toxicology. | |
Molecular Weight |
300.4 g/mol | |
Source | PubChem | |
URL | https://pubchem.ncbi.nlm.nih.gov | |
Description | Data deposited in or computed by PubChem | |
CAS No. |
13488-22-7 | |
Record name | 5,8,11-Eicosatriynoic acid | |
Source | CAS Common Chemistry | |
URL | https://commonchemistry.cas.org/detail?cas_rn=13488-22-7 | |
Description | CAS Common Chemistry is an open community resource for accessing chemical information. Nearly 500,000 chemical substances from CAS REGISTRY cover areas of community interest, including common and frequently regulated chemicals, and those relevant to high school and undergraduate chemistry classes. This chemical information, curated by our expert scientists, is provided in alignment with our mission as a division of the American Chemical Society. | |
Explanation | The data from CAS Common Chemistry is provided under a CC-BY-NC 4.0 license, unless otherwise stated. | |
Record name | 5,8,11-Eicosatriynoic acid | |
Source | ChemIDplus | |
URL | https://pubchem.ncbi.nlm.nih.gov/substance/?source=chemidplus&sourceid=0013488227 | |
Description | ChemIDplus is a free, web search system that provides access to the structure and nomenclature authority files used for the identification of chemical substances cited in National Library of Medicine (NLM) databases, including the TOXNET system. | |
Record name | 5,8,11-Eicosatriynoic acid | |
Source | EPA DSSTox | |
URL | https://comptox.epa.gov/dashboard/DTXSID10159018 | |
Description | DSSTox provides a high quality public chemistry resource for supporting improved predictive toxicology. | |
Record name | 5,8,11-EICOSATRIYNOIC ACID | |
Source | FDA Global Substance Registration System (GSRS) | |
URL | https://gsrs.ncats.nih.gov/ginas/app/beta/substances/R7EU6LY7HJ | |
Description | The FDA Global Substance Registration System (GSRS) enables the efficient and accurate exchange of information on what substances are in regulated products. Instead of relying on names, which vary across regulatory domains, countries, and regions, the GSRS knowledge base makes it possible for substances to be defined by standardized, scientific descriptions. | |
Explanation | Unless otherwise noted, the contents of the FDA website (www.fda.gov), both text and graphics, are not copyrighted. They are in the public domain and may be republished, reprinted and otherwise used freely by anyone without the need to obtain permission from FDA. Credit to the U.S. Food and Drug Administration as the source is appreciated but not required. | |
Retrosynthesis Analysis
AI-Powered Synthesis Planning: Our tool employs the Template_relevance Pistachio, Template_relevance Bkms_metabolic, Template_relevance Pistachio_ringbreaker, Template_relevance Reaxys, Template_relevance Reaxys_biocatalysis model, leveraging a vast database of chemical reactions to predict feasible synthetic routes.
One-Step Synthesis Focus: Specifically designed for one-step synthesis, it provides concise and direct routes for your target compounds, streamlining the synthesis process.
Accurate Predictions: Utilizing the extensive PISTACHIO, BKMS_METABOLIC, PISTACHIO_RINGBREAKER, REAXYS, REAXYS_BIOCATALYSIS database, our tool offers high-accuracy predictions, reflecting the latest in chemical research and data.
Strategy Settings
Precursor scoring | Relevance Heuristic |
---|---|
Min. plausibility | 0.01 |
Model | Template_relevance |
Template Set | Pistachio/Bkms_metabolic/Pistachio_ringbreaker/Reaxys/Reaxys_biocatalysis |
Top-N result to add to graph | 6 |
Feasible Synthetic Routes
Q1: What is the primary mechanism of action of 5,8,11-Eicosatriynoic acid?
A: 5,8,11-Eicosatriynoic acid acts primarily as a mechanism-based inhibitor of lipoxygenases. [] It acts as a suicidal substrate, being converted by the enzyme into a highly reactive allene hydroperoxide. This intermediate then reacts with a methionine residue at the enzyme's active site, leading to irreversible inactivation. []
Q2: Does 5,8,11-Eicosatriynoic acid influence calcium signaling pathways?
A: Yes, research indicates that 5,8,11-Eicosatriynoic acid can induce a concentration-dependent increase in intracellular calcium ([Ca2+]i) in Madin Darby canine kidney cells. This effect is attributed to both the release of calcium from intracellular stores (endoplasmic reticulum, mitochondria, and others) and the influx of extracellular calcium. []
Q3: What is the impact of 5,8,11-Eicosatriynoic acid on cell proliferation?
A: Studies suggest that 5,8,11-Eicosatriynoic acid may play a role in cell proliferation. For instance, it has been shown to block platelet-induced proliferation of human fibroblasts, likely through the inhibition of 5-lipoxygenase and the subsequent reduction of 5-hydroxyeicosatetraenoic acid (5-HETE) production. []
Q4: What is the molecular formula and weight of 5,8,11-Eicosatriynoic acid?
A4: The molecular formula of 5,8,11-Eicosatriynoic acid is C20H28O2. Its molecular weight is 300.44 g/mol.
Q5: Has 5,8,11-Eicosatriynoic acid demonstrated any catalytic properties itself?
A5: While 5,8,11-Eicosatriynoic acid is not known to possess inherent catalytic activity, its presence can influence the catalytic activity of enzymes like lipoxygenase. It acts as a substrate analog that binds to the enzyme's active site, ultimately leading to irreversible inactivation rather than catalytic turnover.
Q6: In what biological systems has 5,8,11-Eicosatriynoic acid shown efficacy in vitro?
A6: 5,8,11-Eicosatriynoic acid has demonstrated efficacy in various in vitro systems. Examples include:
- Inhibition of lipoxygenase activity in cell lysates and purified enzyme preparations: It effectively inhibits lipoxygenase activity in various cell types and tissues, including soybeans, reticulocytes, human term placenta, and rat brain. [, , , ]
- Attenuation of agonist-induced calcium mobilization in platelets: It reduces thrombin- and thromboxane mimetic-induced increases in intracellular calcium in human platelets. []
- Modulation of mitogen responses in lymphocytes: It can synergistically enhance mitogen-induced responses in human lymphocytes when combined with cyclooxygenase inhibitors. [, ]
Q7: Are there other compounds with similar mechanisms of action to 5,8,11-Eicosatriynoic acid?
A7: Yes, several other compounds inhibit lipoxygenase enzymes, including:
- 5,8,11,14-Eicosatetraynoic acid (ETYA): A related acetylenic fatty acid that inhibits both 5-lipoxygenase and 12-lipoxygenase. [, ]
- Nordihydroguaiaretic acid (NDGA): A natural product with potent antioxidant and lipoxygenase inhibitory activity. [, ]
- Baicalein: A flavonoid compound known for its antioxidant and lipoxygenase inhibitory properties. [, ]
- Cinnamyl-3,4-dihydroxy-α-cyanocinnamate (CDC): A selective inhibitor of 5-lipoxygenase. []
試験管内研究製品の免責事項と情報
BenchChemで提示されるすべての記事および製品情報は、情報提供を目的としています。BenchChemで購入可能な製品は、生体外研究のために特別に設計されています。生体外研究は、ラテン語の "in glass" に由来し、生物体の外で行われる実験を指します。これらの製品は医薬品または薬として分類されておらず、FDAから任何の医療状態、病気、または疾患の予防、治療、または治癒のために承認されていません。これらの製品を人間または動物に体内に導入する形態は、法律により厳格に禁止されています。これらのガイドラインに従うことは、研究と実験において法的および倫理的な基準の遵守を確実にするために重要です。