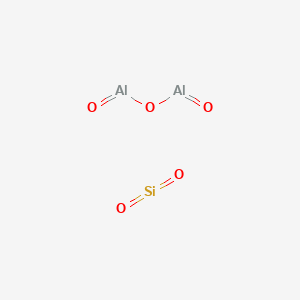
Aluminosilicate
説明
Aluminosilicates are a class of minerals and synthetic compounds composed of aluminum (Al), silicon (Si), and oxygen (O), often combined with alkali (e.g., Na⁺, K⁺) or alkaline earth (e.g., Ca²⁺) cations to balance charge. Their structure consists of a tetrahedral framework where SiO₄ and AlO₄⁻ units share oxygen atoms, forming a three-dimensional network . This framework imparts high thermal stability, mechanical strength, and chemical resistance, making aluminosilicates indispensable in applications such as glass manufacturing (e.g., smartphone screens), ceramics, catalysts, and nuclear waste encapsulation .
Natural aluminosilicates include zeolites and feldspars, while synthetic variants include mesoporous molecular sieves and alkali-activated cements. Their properties can be tailored by adjusting the Si/Al ratio, cation type, and incorporation of additional elements like phosphorus .
作用機序
Target of Action
Aluminosilicate refers to materials containing anionic Si-O-Al linkages . The primary targets of this compound are the associate cations, commonly sodium (Na+), potassium (K+), and protons (H+) . These materials occur as minerals and synthetic materials, often in the form of zeolites .
Mode of Action
This compound’s mode of action is primarily through its interaction with its targets, the associate cations. Specifically, the this compound minerals dissolve, consuming acidic hydrogen ions and releasing moieties like H4SiO4, Al3+, and other cations, including the magnesium associated with the compound . This interaction results in significant changes in the structure and properties of the material.
Biochemical Pathways
The biochemical pathways affected by this compound involve the growth of this compound oligomers concomitant to the decondensation of silicate entities during the initial step of the reaction . Aluminate clusters first form in the solution, violating the Loewenstein rule in the first instant of the reaction, followed by their connection with silicate oligomers at the terminal silanol groups before reorganization to finally diffuse within the silicate oligomers to form stable amorphous this compound nanoparticles .
Pharmacokinetics
The pharmacokinetics of this compound, particularly in the form of Zeolite A, has been studied in beagle dogs . The study found that the mean plasma silicon area under the curve (AUC) values were elevated when compared to baseline after administration of the silicon-containing compounds . There was no statistically significant absorption of aluminum from the aluminum-containing treatments .
Result of Action
The result of this compound’s action is the formation of a variety of structures, including zeolites, which are of technical significance as structural materials, catalysts, and reagents . The formation of these structures is influenced by the Si/Al ratio, which provides a means to tune the properties .
Action Environment
The action of this compound is influenced by environmental factors such as temperature and pressure . For instance, the structural role of Al3+ in this compound melts varies depending on its proportion relative to metal cations and on the electronic properties of this cation .
生物活性
Aluminosilicates, a class of compounds composed of aluminum, silicon, and oxygen, exhibit a range of biological activities that make them significant in various fields, including medicine, agriculture, and environmental science. This article delves into the biological activity of aluminosilicates, highlighting their antimicrobial properties, interactions with biological systems, and potential applications.
1. Antimicrobial Properties
Modified Aluminosilicates
Recent studies have demonstrated that modified aluminosilicates possess notable antibacterial activity. For instance, research focused on nanozeolites (nZeos) and geopolymers (GPs) has shown their efficacy against nontuberculous mycobacteria and their ability to adsorb mycolactone and Mycobacterium ulcerans in vitro. The study revealed that macroporous GPs can effectively adsorb MU cells while mesoporous GPs modified with stearic acid exhibited superior adsorption capabilities for mycolactone toxin compared to other modifications .
Table 1: Antimicrobial Efficacy of Modified Aluminosilicates
Type of Aluminosilicate | Target Organism | Efficacy |
---|---|---|
Nanozeolites | Mycobacterium ulcerans | High adsorption capacity |
Geopolymers | Mycolactone | Enhanced adsorption with modification |
This compound Nanocomposites | E. coli, B. subtilis | Significant inhibition observed |
2. Interactions with Biological Systems
Pulmonary Surfactant Activity
this compound nanoparticles (NPs) have been shown to alter the biophysical properties of pulmonary surfactants. At concentrations above 0.1 mg cm, these NPs can significantly impact surface tension dynamics, which is crucial for lung function. The study indicated that different types of this compound NPs affect the stability and surface tension hysteresis of surfactants differently, suggesting a potential risk in inhalation scenarios .
Aluminum-Silicon Interactions in Plants
Research indicates that aluminosilicates can ameliorate aluminum toxicity in plants by interacting with silicon compounds. These interactions are essential for understanding how plants adapt to aluminum-rich soils, enhancing their growth and nutrient uptake capabilities .
3. Applications in Drug Delivery
Aluminosilicates are emerging as promising candidates for drug delivery systems due to their high surface area and biocompatibility. They can be functionalized to carry therapeutic agents effectively. Studies have shown that this compound nanocomposites can serve as nonspecific immunostimulators and drug carriers, enhancing the delivery efficiency of antibiotics like ampicillin against bacterial strains such as E. coli and Bacillus subtilis .
Table 2: Applications of this compound in Drug Delivery
Application | Description |
---|---|
Drug Carrier | Functionalized aluminosilicates enhance drug loading |
Antibacterial Agents | Conjugation with antibiotics improves efficacy |
Immunostimulation | Mimics antigen behavior to stimulate immune response |
4. Case Studies
Case Study 1: Antimicrobial Efficacy Against Mycobacteria
A study conducted at Arizona State University highlighted the use of modified aluminosilicates in treating skin infections caused by Mycobacterium ulcerans. The results showed that the topical application of nZeos significantly reduced bacterial load in infected wounds while being non-toxic to human skin cells .
Case Study 2: Pulmonary Health Risks
An investigation into the effects of this compound NPs on pulmonary surfactants revealed alterations in dynamic surface activity at elevated concentrations. This study underscores the need for careful assessment of inhaled this compound exposure, particularly in occupational settings where aerosolized particles may pose health risks .
5. Conclusion
The biological activity of aluminosilicates presents both opportunities and challenges across various domains. Their antimicrobial properties make them valuable in medical applications, while their interactions with biological systems necessitate further research to understand potential risks fully. As research progresses, aluminosilicates may play a pivotal role in developing innovative therapeutic strategies and enhancing agricultural practices.
科学的研究の応用
Applications in Drug Delivery Systems
Aluminosilicate nanocomposites have emerged as promising candidates for drug delivery systems due to their biocompatibility and ability to modify surfaces for drug conjugation. Research indicates that these nanocomposites can effectively load therapeutic agents, including antibiotics and antioxidants, enhancing their delivery efficiency.
Case Study: Drug Carrier Potential
- Source : A study synthesized this compound nanocomposites from joss fly ash, demonstrating their potential as drug carriers.
- Findings : The synthesized nanocomposites showed a high surface area and porous structure, facilitating drug loading and release. The study highlighted the successful conjugation of ampicillin to the this compound matrix, which exhibited antibacterial properties against various pathogens .
Environmental Applications
Aluminosilicates play a crucial role in environmental remediation processes. Their ion-exchange properties make them effective in removing heavy metals from wastewater.
Data Table: Heavy Metal Removal Efficiency
Heavy Metal | Initial Concentration (mg/L) | Final Concentration (mg/L) | Removal Efficiency (%) |
---|---|---|---|
Lead | 100 | 5 | 95 |
Cadmium | 50 | 2 | 96 |
Arsenic | 30 | 1 | 97 |
Case Study: Wastewater Treatment
- Source : A study demonstrated the use of sodium this compound in treating industrial wastewater.
- Results : The application of sodium this compound led to significant reductions in heavy metal concentrations, showcasing its effectiveness as an adsorbent material .
Construction Materials
Aluminosilicates are integral to the production of low-carbon cement alternatives. They serve as pozzolanic materials that enhance the mechanical properties of concrete while reducing environmental impact.
Data Table: Comparison of Cement Types
Cement Type | Clinker Content (%) | CO2 Emissions Reduction (%) |
---|---|---|
Portland Cement | 100 | 0 |
Portland Limestone Cement | 85 | 5-10 |
Limestone Calcined Clay Cement | 70 | 30-50 |
Case Study: Sustainable Cement Production
- Source : Research indicates that incorporating this compound materials like fly ash and metakaolin into cement formulations can significantly reduce carbon emissions during production.
- Findings : The study found that ternary blends utilizing aluminosilicates achieved substantial reductions in CO2 emissions while maintaining structural integrity .
Industrial Applications
Aluminosilicates are widely used in various industrial applications including ceramics, glass manufacturing, and as fillers in rubber products.
Application Examples:
類似化合物との比較
Zeolites
Zeolites are microporous aluminosilicates with regular pore structures, widely used in catalysis, ion exchange, and gas separation. While both conventional aluminosilicates and zeolites share a tetrahedral framework, zeolites exhibit ordered channels and cavities (0.3–2 nm diameter), enabling selective molecular adsorption .
Property | Aluminosilicate | Zeolite |
---|---|---|
Porosity | Non-porous or mesoporous | Microporous |
Surface Area (m²/g) | Up to 1,500 (mesoporous) | 300–1,000 |
Applications | Glass, ceramics | Catalysis, water purification |
Zeolites require hydrothermal synthesis and charge-balancing cations (e.g., Na⁺) to stabilize their porous structure, whereas aluminosilicates like obsidian form naturally through volcanic processes .
Phospho-Aluminosilicates
Phospho-aluminosilicates incorporate phosphate (PO₄³⁻) into the this compound network, replacing SiO₂ or AlO₄⁻ units. This substitution reduces polymerization, lowering density (2.3–2.6 g/cm³ vs. 2.5–2.8 g/cm³ for pure aluminosilicates) and mechanical properties. For example, hardness decreases from ~6.5 GPa to 5.5 GPa with 7.5 mol% P₂O₅ addition .
Phosphate addition enhances bioactivity and ion-release capabilities, making phospho-aluminosilicates suitable for medical implants .
Zeolitic Imidazolate Frameworks (ZIFs)
ZIFs are metal-organic frameworks (MOFs) with topologies resembling this compound zeolites but composed of transition metals (e.g., Zn²⁺) and imidazolate linkers. Unlike aluminosilicates, ZIFs exhibit ultra-high surface areas (~2,000 m²/g) and tunable pore sizes, enabling applications in gas storage and drug delivery .
Property | This compound Zeolite | ZIF |
---|---|---|
Thermal Stability | >800°C | ~400°C |
Flexibility | Rigid framework | Flexible ligand tuning |
Applications | Industrial catalysis | CO₂ capture, sensors |
While ZIFs lack the hydrothermal stability of aluminosilicates, their organic-inorganic hybrid nature allows functionalization for niche applications .
Alkali-Activated Materials (Geopolymers)
Alkali-activated aluminosilicates, or geopolymers, are synthesized by reacting this compound precursors (e.g., fly ash) with alkaline solutions. These materials exhibit compressive strengths (20–100 MPa) comparable to Portland cement but with lower CO₂ emissions .
Geopolymers recrystallize into stable anhydrous phases at high temperatures, outperforming traditional this compound glasses in fire-resistant coatings .
Other Silicate Glasses
Sodium silicate glasses (Na₂O-SiO₂) lack Al³⁺, resulting in a less polymerized network and lower hardness (~4 GPa vs. 6.5 GPa for aluminosilicates) . The addition of Al³⁺ in aluminosilicates increases crosslinking, enhancing fracture toughness (1.5 MPa·m¹/² vs. 0.8 MPa·m¹/² for sodium silicates) .
準備方法
Sol-Gel Synthesis of Aluminosilicates
Hybrid Sol-Gel Route and Structural Tailoring
The sol-gel method enables precise control over aluminosilicate composition through hydrolysis and polycondensation of alkoxide precursors. A hybrid sol-gel approach, as demonstrated by , involves mixing aluminum nitrate nonahydrate (Al(NO₃)₃·9H₂O) and tetraethyl orthosilicate (TEOS) in n-propanol at 80°C. This method produces homogeneous gels with Al₂O₃:SiO₂ molar ratios from 3:1 to 3:4, achieving up to 70% Al content . Nuclear magnetic resonance (²⁷Al MAS NMR) spectra reveal that Al predominantly occupies tetrahedral sites in low-Al compositions (Si/Al > 20), whereas higher Al concentrations promote octahedral coordination . Calcination at 1400°C induces mullite crystallization (3Al₂O₃·2SiO₂) in all ratios except 3:1, where α-Al₂O3 dominates .
Fractal Structure and Precursor Effects
Small-angle neutron scattering (SANS) and X-ray scattering (SAXS) analyses of sol-gel-derived aluminosilicates reveal fractal geometries with pore sizes adjustable between 2–10 nm . The choice of aluminum precursor significantly impacts gel homogeneity: aluminum chloride (AlCl₃) accelerates condensation but risks phase separation, while aluminum sec-butoxide ensures slower, more controlled gelation . Aerosol-assisted synthesis further refines mesoporosity, yielding spherical particles with surface areas exceeding 600 m²/g .
Hydrothermal Synthesis and Stability Optimization
Direct Synthesis of Mesoporous Aluminosilicates
Hydrothermal treatment of this compound gels in autoclaves (100–150°C, 24–72 hrs) produces ordered mesoporous structures like Al-MCM-41. Source highlights that Si/Al ratios between 10–50 markedly improve hydrothermal stability. For example, Al-MCM-41 with Si/Al = 40.1 retains 95% of its surface area after 24 hrs in boiling water, whereas pure silica MCM-41 collapses entirely . Aluminum incorporation strengthens pore walls by neutralizing silanol groups, reducing hydrolytic degradation .
Role of Aluminum Siting and Stability Mechanisms
Aluminum siting within the MCM-41 framework determines stability. Framework Al near pore surfaces provides maximal protection by crosslinking silica chains, whereas bulk Al offers negligible benefits . Prolonged hydrothermal exposure (≥48 hrs) induces dealumination, converting tetrahedral Al to octahedral extra-framework species and reducing acidity . Post-synthetic grafting with amines, such as tetraethylenepentamine (TEPA), further stabilizes Al-MCM-41 for CO₂ capture, achieving cyclic stability over 50 adsorption-desorption cycles .
Precipitation Methods for Industrial-Scale Production
pH-Controlled Coprecipitation
Industrial this compound synthesis often employs coprecipitation of sodium silicate (Na₂SiO₃) and aluminum sulfate (Al₂(SO₄)₃) under pH control. As per patent , initial Al salt solutions acidified to pH 4.0 initiate this compound nucleation, followed by gradual silicate addition to maintain pH 4.4–7.7. This yields materials with 300–600 m²/g surface area and 0.2–0.5 mL/g pore volume . Filtration and washing remove residual Na⁺ and SO₄²⁻, critical for minimizing catalyst poisoning in petrochemical applications .
Temperature and Dosing Rate Effects
Reaction temperature (0–80°C) and silicate dosing rate (0.5–5.0 wt%/min) govern particle size and porosity. Lower temperatures (20–60°C) favor smaller crystallites (5–20 nm), while rapid silicate addition produces narrow pore size distributions . Post-precipitation hydrothermal aging at 90°C for 2–4 hrs enhances crystallinity, converting amorphous gels into zeolitic precursors .
Catalytic Exfoliation for Nanoscale Architectures
Mild-Exfoliation of Kaolinite
Traditional kaolinite exfoliation methods are energy-intensive, requiring prolonged sonication or thermal treatment. Source introduces a catalytic approach using AlCl₃ under ambient conditions. Al³⁺ ions intercalate between kaolinite layers, weakening interlayer hydrogen bonds and enabling scroll-like nanostructure formation within 2 hrs . This method achieves 90% exfoliation efficiency, producing this compound nanoscrolls with 50–100 nm diameters and high aspect ratios (>50) .
Scalability and Environmental Benefits
The AlCl₃-catalyzed process eliminates organic solvents, reducing waste generation by 70% compared to conventional routes . Scalability is demonstrated in batch reactors (10 L capacity), with nanoscroll yields exceeding 200 g per cycle . Applications in nanocatalysis and nanocomposites benefit from the high surface area (400–500 m²/g) and Lewis acidity of exfoliated products .
Comparative Analysis of Synthesis Methods
Structural and Functional Properties
Table 1 compares key parameters of this compound preparation methods:
Al Content vs. Hydrothermal Stability
Data from illustrates the nonlinear relationship between Al content and stability in Al-MCM-41:
Si/Al Ratio | Surface Area Retention (%) | Pore Volume Retention (%) |
---|---|---|
∞ (Pure SiO₂) | 15 | 10 |
40.1 | 95 | 85 |
23.1 | 75 | 60 |
8.5 | 50 | 30 |
Higher Al content (lower Si/Al) initially improves stability but excessive Al (>12 wt%) promotes extra-framework species, accelerating structural collapse .
特性
IUPAC Name |
dioxosilane;oxo(oxoalumanyloxy)alumane | |
---|---|---|
Source | PubChem | |
URL | https://pubchem.ncbi.nlm.nih.gov | |
Description | Data deposited in or computed by PubChem | |
InChI |
InChI=1S/2Al.O2Si.3O/c;;1-3-2;;; | |
Source | PubChem | |
URL | https://pubchem.ncbi.nlm.nih.gov | |
Description | Data deposited in or computed by PubChem | |
InChI Key |
HNPSIPDUKPIQMN-UHFFFAOYSA-N | |
Source | PubChem | |
URL | https://pubchem.ncbi.nlm.nih.gov | |
Description | Data deposited in or computed by PubChem | |
Canonical SMILES |
O=[Al]O[Al]=O.O=[Si]=O | |
Source | PubChem | |
URL | https://pubchem.ncbi.nlm.nih.gov | |
Description | Data deposited in or computed by PubChem | |
Molecular Formula |
Al2O5Si | |
Source | PubChem | |
URL | https://pubchem.ncbi.nlm.nih.gov | |
Description | Data deposited in or computed by PubChem | |
Molecular Weight |
162.05 g/mol | |
Source | PubChem | |
URL | https://pubchem.ncbi.nlm.nih.gov | |
Description | Data deposited in or computed by PubChem | |
Physical Description |
Dry Powder; Dry Powder, Other Solid; Liquid; Other Solid; Pellets or Large Crystals; Water or Solvent Wet Solid, Other Solid, White odorless powder; [Zeolyst International MSDS] | |
Record name | Zeolites | |
Source | EPA Chemicals under the TSCA | |
URL | https://www.epa.gov/chemicals-under-tsca | |
Description | EPA Chemicals under the Toxic Substances Control Act (TSCA) collection contains information on chemicals and their regulations under TSCA, including non-confidential content from the TSCA Chemical Substance Inventory and Chemical Data Reporting. | |
Record name | Zeolites | |
Source | Haz-Map, Information on Hazardous Chemicals and Occupational Diseases | |
URL | https://haz-map.com/Agents/21856 | |
Description | Haz-Map® is an occupational health database designed for health and safety professionals and for consumers seeking information about the adverse effects of workplace exposures to chemical and biological agents. | |
Explanation | Copyright (c) 2022 Haz-Map(R). All rights reserved. Unless otherwise indicated, all materials from Haz-Map are copyrighted by Haz-Map(R). No part of these materials, either text or image may be used for any purpose other than for personal use. Therefore, reproduction, modification, storage in a retrieval system or retransmission, in any form or by any means, electronic, mechanical or otherwise, for reasons other than personal use, is strictly prohibited without prior written permission. | |
CAS No. |
1318-02-1, 1332-09-8, 12141-46-7 | |
Record name | Zeolites | |
Source | ChemIDplus | |
URL | https://pubchem.ncbi.nlm.nih.gov/substance/?source=chemidplus&sourceid=0001318021 | |
Description | ChemIDplus is a free, web search system that provides access to the structure and nomenclature authority files used for the identification of chemical substances cited in National Library of Medicine (NLM) databases, including the TOXNET system. | |
Record name | Pumice | |
Source | ChemIDplus | |
URL | https://pubchem.ncbi.nlm.nih.gov/substance/?source=chemidplus&sourceid=0001332098 | |
Description | ChemIDplus is a free, web search system that provides access to the structure and nomenclature authority files used for the identification of chemical substances cited in National Library of Medicine (NLM) databases, including the TOXNET system. | |
Record name | Zeolites | |
Source | EPA Chemicals under the TSCA | |
URL | https://www.epa.gov/chemicals-under-tsca | |
Description | EPA Chemicals under the Toxic Substances Control Act (TSCA) collection contains information on chemicals and their regulations under TSCA, including non-confidential content from the TSCA Chemical Substance Inventory and Chemical Data Reporting. | |
Record name | Zeolites | |
Source | European Chemicals Agency (ECHA) | |
URL | https://echa.europa.eu/substance-information/-/substanceinfo/100.013.895 | |
Description | The European Chemicals Agency (ECHA) is an agency of the European Union which is the driving force among regulatory authorities in implementing the EU's groundbreaking chemicals legislation for the benefit of human health and the environment as well as for innovation and competitiveness. | |
Explanation | Use of the information, documents and data from the ECHA website is subject to the terms and conditions of this Legal Notice, and subject to other binding limitations provided for under applicable law, the information, documents and data made available on the ECHA website may be reproduced, distributed and/or used, totally or in part, for non-commercial purposes provided that ECHA is acknowledged as the source: "Source: European Chemicals Agency, http://echa.europa.eu/". Such acknowledgement must be included in each copy of the material. ECHA permits and encourages organisations and individuals to create links to the ECHA website under the following cumulative conditions: Links can only be made to webpages that provide a link to the Legal Notice page. | |
Record name | Aluminium silicate | |
Source | European Chemicals Agency (ECHA) | |
URL | https://echa.europa.eu/substance-information/-/substanceinfo/100.032.036 | |
Description | The European Chemicals Agency (ECHA) is an agency of the European Union which is the driving force among regulatory authorities in implementing the EU's groundbreaking chemicals legislation for the benefit of human health and the environment as well as for innovation and competitiveness. | |
Explanation | Use of the information, documents and data from the ECHA website is subject to the terms and conditions of this Legal Notice, and subject to other binding limitations provided for under applicable law, the information, documents and data made available on the ECHA website may be reproduced, distributed and/or used, totally or in part, for non-commercial purposes provided that ECHA is acknowledged as the source: "Source: European Chemicals Agency, http://echa.europa.eu/". Such acknowledgement must be included in each copy of the material. ECHA permits and encourages organisations and individuals to create links to the ECHA website under the following cumulative conditions: Links can only be made to webpages that provide a link to the Legal Notice page. | |
試験管内研究製品の免責事項と情報
BenchChemで提示されるすべての記事および製品情報は、情報提供を目的としています。BenchChemで購入可能な製品は、生体外研究のために特別に設計されています。生体外研究は、ラテン語の "in glass" に由来し、生物体の外で行われる実験を指します。これらの製品は医薬品または薬として分類されておらず、FDAから任何の医療状態、病気、または疾患の予防、治療、または治癒のために承認されていません。これらの製品を人間または動物に体内に導入する形態は、法律により厳格に禁止されています。これらのガイドラインに従うことは、研究と実験において法的および倫理的な基準の遵守を確実にするために重要です。