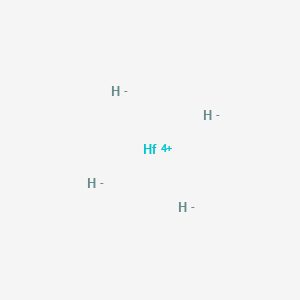
Hafnium tetrahydride
- 専門家チームからの見積もりを受け取るには、QUICK INQUIRYをクリックしてください。
- 品質商品を競争力のある価格で提供し、研究に集中できます。
説明
Hafnium tetrahydride is a chemical compound composed of hafnium and hydrogen atoms It is represented by the chemical formula HfH₄
準備方法
Synthetic Routes and Reaction Conditions: Hafnium tetrahydride can be synthesized through the direct reaction of hafnium metal with hydrogen gas. The reaction typically occurs at elevated temperatures and pressures to facilitate the formation of the hydride. The general reaction is as follows: [ \text{Hf} + 2\text{H}_2 \rightarrow \text{HfH}_4 ]
Industrial Production Methods: Industrial production of this compound involves the use of high-purity hafnium metal and controlled hydrogenation processes. The reaction conditions, such as temperature and pressure, are optimized to ensure maximum yield and purity of the product. The process may also involve purification steps to remove any impurities or by-products.
化学反応の分析
Types of Reactions: Hafnium tetrahydride undergoes various chemical reactions, including:
Oxidation: this compound can react with oxygen to form hafnium oxide and water. [ \text{HfH}_4 + 2\text{O}_2 \rightarrow \text{HfO}_2 + 2\text{H}_2\text{O} ]
Reduction: It can be reduced to hafnium metal and hydrogen gas under certain conditions. [ \text{HfH}_4 \rightarrow \text{Hf} + 2\text{H}_2 ]
Substitution: this compound can undergo substitution reactions with halogens to form hafnium halides and hydrogen gas. [ \text{HfH}_4 + 4\text{X}_2 \rightarrow \text{HfX}_4 + 4\text{H}_2 ] (where X represents a halogen such as chlorine, bromine, or iodine)
Common Reagents and Conditions:
Oxidation: Oxygen or air at elevated temperatures.
Reduction: High temperatures and a reducing atmosphere (e.g., hydrogen gas).
Substitution: Halogen gases (e.g., chlorine, bromine) at elevated temperatures.
Major Products Formed:
Oxidation: Hafnium oxide (HfO₂) and water (H₂O).
Reduction: Hafnium metal (Hf) and hydrogen gas (H₂).
Substitution: Hafnium halides (e.g., HfCl₄) and hydrogen gas (H₂).
科学的研究の応用
Hafnium tetrahydride has several scientific research applications, including:
Materials Science: It is used in the development of advanced materials with unique properties, such as high-temperature stability and resistance to corrosion.
Nuclear Technology: this compound is explored for its potential use as a neutron moderator and absorber in nuclear reactors due to its high neutron absorption cross-section.
Catalysis: It serves as a catalyst in various chemical reactions, including hydrogenation and dehydrogenation processes.
Hydrogen Storage: this compound is investigated for its potential use in hydrogen storage systems due to its ability to release hydrogen gas upon decomposition.
作用機序
The mechanism by which hafnium tetrahydride exerts its effects involves the interaction of hafnium atoms with hydrogen atoms. The hydrogen atoms are bonded to the hafnium atom, forming a stable hydride. The compound can release hydrogen gas upon decomposition, making it useful in hydrogen storage applications. Additionally, the high neutron absorption cross-section of hafnium makes this compound effective in nuclear applications.
類似化合物との比較
Hafnium tetrahydride can be compared with other similar compounds, such as:
Titanium tetrahydride (TiH₄): Similar to this compound, titanium tetrahydride is used in hydrogen storage and catalysis. this compound has a higher neutron absorption cross-section, making it more suitable for nuclear applications.
Zirconium tetrahydride (ZrH₄): Zirconium tetrahydride is also used in hydrogen storage and nuclear technology. This compound is preferred in certain applications due to its higher neutron absorption capability.
Group 14 Tetrahydrides: Compounds such as methane (CH₄), silane (SiH₄), and germane (GeH₄) are also tetrahydrides but differ significantly in their chemical properties and applications. This compound is unique due to its metallic nature and high neutron absorption cross-section.
生物活性
Hafnium tetrahydride (HfH4) is a compound of hafnium that has garnered interest due to its unique properties and potential applications in various fields, including materials science and medicine. However, research specifically focusing on the biological activity of this compound is limited. This article compiles available data regarding its biological implications, particularly in the context of its interactions with biological systems.
Overview of Hafnium and Its Compounds
Hafnium (Hf) is a transition metal that shares similarities with zirconium and is primarily used in nuclear reactors and as a refractory material. While hafnium itself has been studied for its properties and applications, its biological activity remains underexplored. Recent studies have indicated that hafnium compounds, including hafnium oxide and hafnium disulfide, show promising biomedical applications such as osteogenic potential and anti-inflammatory effects .
Toxicological Assessments
Initial assessments of the biological effects of hafnium tetrafluoride (HfF4), a related compound, have been conducted to evaluate its toxicity following intratracheal instillation in rats. These studies suggest that hafnium compounds can induce pulmonary inflammation, which may be relevant when considering the safety profile of this compound . The specific biological activity of HfH4 has not been extensively characterized, but insights from related compounds provide a foundation for understanding potential biological interactions.
Case Studies and Research Findings
- Osteogenic Potential : A systematic review highlighted the osteogenic properties of hafnium compounds, showing that they can promote bone tissue regeneration. For instance, studies demonstrated that hafnium oxide films enhanced cell viability and proliferation in human osteoblast-like cells . Although HfH4 was not directly tested, the positive results from other hafnium compounds suggest a potential for similar biological activity.
- Anti-inflammatory Effects : Hafnium disulfide nanoparticles have been evaluated for their therapeutic effects on inflammatory bowel disease in animal models. These studies indicated significant healing effects on intestinal mucosal barriers and reductions in pro-inflammatory markers . While HfH4 has not been tested in this context, the anti-inflammatory properties observed in other hafnium compounds may be indicative of potential benefits.
Data Table: Summary of Biological Studies on Hafnium Compounds
Study Reference | Compound Studied | Findings | Model Used |
---|---|---|---|
Li et al., 2022 | Hafnium disulfide | Therapeutic effects on acute colitis; repair of intestinal barrier | NCM460 cells, Balb/c mice |
Fohlerova et al., 2019 | Hafnium oxide | Enhanced cell viability and attachment; promotes osteoblast proliferation | MG-63 cells |
Miyazaki et al., 2018 | Pure Hf and Ti-Hf alloys | Apatite-forming abilities; bone-bonding potential | In vitro assays |
Future Directions
The exploration of this compound's biological activity requires further research to elucidate its interactions with biological systems. Given the promising findings related to other hafnium compounds, future studies should focus on:
- In vitro Studies : Assessing the cytotoxicity and biocompatibility of HfH4 using various cell lines.
- In vivo Models : Conducting animal studies to evaluate systemic effects following exposure to HfH4.
- Mechanistic Investigations : Understanding the molecular pathways involved in any observed biological activities or toxicities.
特性
IUPAC Name |
hafnium(4+);hydride |
Source
|
---|---|---|
Source | PubChem | |
URL | https://pubchem.ncbi.nlm.nih.gov | |
Description | Data deposited in or computed by PubChem | |
InChI |
InChI=1S/Hf.4H/q+4;4*-1 |
Source
|
Source | PubChem | |
URL | https://pubchem.ncbi.nlm.nih.gov | |
Description | Data deposited in or computed by PubChem | |
InChI Key |
OSXSTNIHZFKNLY-UHFFFAOYSA-N |
Source
|
Source | PubChem | |
URL | https://pubchem.ncbi.nlm.nih.gov | |
Description | Data deposited in or computed by PubChem | |
Canonical SMILES |
[H-].[H-].[H-].[H-].[Hf+4] |
Source
|
Source | PubChem | |
URL | https://pubchem.ncbi.nlm.nih.gov | |
Description | Data deposited in or computed by PubChem | |
Molecular Formula |
H4Hf |
Source
|
Source | PubChem | |
URL | https://pubchem.ncbi.nlm.nih.gov | |
Description | Data deposited in or computed by PubChem | |
DSSTOX Substance ID |
DTXSID40622063 |
Source
|
Record name | Hafnium tetrahydride | |
Source | EPA DSSTox | |
URL | https://comptox.epa.gov/dashboard/DTXSID40622063 | |
Description | DSSTox provides a high quality public chemistry resource for supporting improved predictive toxicology. | |
Molecular Weight |
182.52 g/mol |
Source
|
Source | PubChem | |
URL | https://pubchem.ncbi.nlm.nih.gov | |
Description | Data deposited in or computed by PubChem | |
CAS No. |
12656-74-5 |
Source
|
Record name | Hafnium tetrahydride | |
Source | EPA DSSTox | |
URL | https://comptox.epa.gov/dashboard/DTXSID40622063 | |
Description | DSSTox provides a high quality public chemistry resource for supporting improved predictive toxicology. | |
試験管内研究製品の免責事項と情報
BenchChemで提示されるすべての記事および製品情報は、情報提供を目的としています。BenchChemで購入可能な製品は、生体外研究のために特別に設計されています。生体外研究は、ラテン語の "in glass" に由来し、生物体の外で行われる実験を指します。これらの製品は医薬品または薬として分類されておらず、FDAから任何の医療状態、病気、または疾患の予防、治療、または治癒のために承認されていません。これらの製品を人間または動物に体内に導入する形態は、法律により厳格に禁止されています。これらのガイドラインに従うことは、研究と実験において法的および倫理的な基準の遵守を確実にするために重要です。