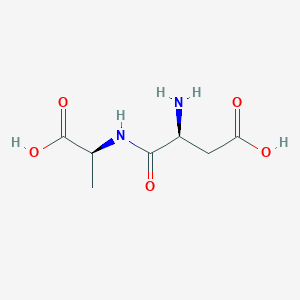
Aspartylalanine
概要
説明
Aspartylalanine (CAS 13433-02-8), also known as L-α-aspartyl-L-alanine, is a dipeptide composed of aspartic acid and alanine residues linked via an α-peptide bond . Its molecular formula is C₇H₁₂N₂O₅, with a molecular weight of 204.18 g/mol. This compound is of interest in biochemistry due to its role as a structural analog of naturally occurring γ-glutamyl peptides and β-aspartyl derivatives. Unlike γ-glutamyl compounds, which are common in metabolic pathways, this compound’s α-configuration influences its stability and solvation properties in aqueous environments .
準備方法
Synthetic Routes and Reaction Conditions: Aspartylalanine can be synthesized through various methods, including both chemical and enzymatic routes. One common method involves the condensation of aspartic acid with alanine under specific reaction conditions. The reaction typically requires a coupling agent, such as carbodiimide, to facilitate the formation of the peptide bond.
Industrial Production Methods: In industrial settings, the production of this compound often involves the use of protecting groups to prevent unwanted side reactions. For example, the amino group of aspartic acid can be protected using carbobenzoxy or formyl groups. The protected aspartic acid is then reacted with alanine methyl ester, followed by the removal of the protective groups to yield this compound .
化学反応の分析
Oxidation Reactions
- Description: Aspartylalanine can undergo oxidation reactions, which typically result in the formation of oxo derivatives.
- Common Reagents: Oxidizing agents such as hydrogen peroxide (H₂O₂) and potassium permanganate (KMnO₄) are commonly used to facilitate oxidation.
- Products: The specific oxo derivatives produced depend on the reaction conditions and the oxidizing agent used.
Reduction Reactions
- Description: Reduction reactions can convert this compound into its reduced forms.
- Common Reagents: Reducing agents like sodium borohydride (NaBH₄) are often employed.
- Products: The products of reduction reactions are reduced forms of this compound, with the exact structure depending on the reducing agent and reaction conditions.
Substitution Reactions
- Description: Substitution reactions can occur at the amino or carboxyl groups of this compound, leading to the formation of different derivatives.
- Common Reagents: Reagents such as acyl chlorides and alkyl halides are used for substitution reactions.
- Products: These reactions yield substituted dipeptides, with the specific product determined by the nature of the acyl or alkyl group introduced.
Hydrolysis
- This compound, being a dipeptide, is susceptible to hydrolysis, which breaks it down into its constituent amino acids, aspartic acid, and alanine.
- Hydrolysis can occur under acidic or basic conditions, or can be catalyzed by enzymes .
Involvement in Biochemical Pathways
- This compound is involved in the aspartate pathway, which is significant in the synthesis of β-alanine.
- It participates in the metabolism of asparagine, an important amino acid in mammals, which acts as a major form of nitrogen storage and transport to sink tissues.
Degradation
- Aspartyl and asparaginyl residues can undergo nonenzymatic degradation in proteins through deamidation, isomerization, and racemization reactions .
- These reactions can alter protein structure and function, with the peptide L-Val-L-Tyr-L-Pro-L-Asn-Gly-L-Ala undergoing rapid deamidation to form an aspartyl succinimide product .
Reactions in Biomolecular Condensates
科学的研究の応用
Biochemistry
Aspartylalanine serves as a model substrate for studying peptide bond formation and cleavage by enzymes such as peptidases. Understanding these interactions is crucial for drug development and protein research. It can also be used to investigate enzyme kinetics, particularly the activity of β-aspartyl peptidase, which catalyzes the hydrolysis of β-aspartyl peptides.
Neuroscience
In neuroscience, this compound is studied for its role in synaptic transmission. It has been shown to increase the amplitude of miniature endplate potentials (MEPPs) in phrenic nerve-diaphragm preparations from rats, suggesting its involvement in neurotransmission and neural signaling. This property highlights its potential therapeutic applications in neurological disorders characterized by impaired neurotransmission.
Pharmacology
Research is ongoing to explore the therapeutic potential of this compound in treating various neurological conditions. Its ability to enhance synaptic transmission positions it as a candidate for developing treatments aimed at improving cognitive functions or alleviating symptoms of neurodegenerative diseases.
Synthetic Applications
This compound can be utilized as a building block in the design of synthetic peptides that mimic specific protein-protein interaction motifs essential for cellular signaling. This application underscores its significance in developing novel therapeutic strategies and understanding protein interactions within biological systems.
Material Science
The compound's properties may lead to innovative applications in creating biomaterials for drug delivery systems. Its ability to form hydrogen bonds allows it to self-organize into ordered structures, which can be utilized in designing self-assembling hydrogels.
Metabolomic Profiling
A study on BALB/c macrophages infected with Leishmania demonstrated alterations in amino acid metabolism, including changes associated with L-arginine levels that could be influenced by dipeptides like this compound. The findings suggest that understanding these interactions may help identify new drug targets for controlling infections.
Synthetic Applications
Recent investigations have explored the use of this compound in synthetic peptides designed to mimic specific protein interaction motifs crucial for cellular signaling. This application underscores its significance in developing novel therapeutic strategies and understanding protein interactions within biological systems.
作用機序
Aspartylalanine exerts its effects by enhancing synaptic transmission in the brain. It increases the amplitude of miniature endplate potentials (MEPPs) in nerve-diaphragm preparations, which suggests its role in modulating neurotransmitter release . The exact molecular targets and pathways involved are still under investigation, but it is believed to interact with specific receptors and ion channels in the nervous system.
類似化合物との比較
Comparison with Structurally Similar Compounds
γ-Glutamyl Peptides
γ-Glutamyl peptides, such as γ-glutamylglutamine and γ-glutamyltaurine, feature a γ-peptide bond between glutamic acid and other amino acids. These compounds are prevalent in redox regulation and neurotransmitter synthesis. Key differences include:
- Functional Implications : The γ-bond in glutamyl peptides allows for greater conformational flexibility, enhancing their interaction with enzymes like γ-glutamyl transpeptidase. This compound’s α-bond restricts its metabolic utility, rendering it less abundant in vivo .
β-Aspartyl Derivatives
β-Aspartyl compounds, such as β-aspartylglycine and β-aspartyltaurine, are isomers of this compound with peptide bonds at the β-carboxyl group of aspartic acid. These derivatives exhibit distinct biochemical behaviors:
- Structural Notes: β-Aspartyl bonds are less common in nature due to steric hindrance but are observed in aged proteins or under oxidative stress .
Aspartylphenylalanine and Derivatives
Aspartylphenylalanine (e.g., L-α-aspartyl-L-phenylalanine methyl ester, or aspartame) shares structural homology with this compound but differs in functional applications:
- Key Contrast : The phenylalanine residue and methyl ester in aspartame drastically alter its taste receptor affinity compared to this compound, which lacks sweetening properties .
This compound in Larger Peptides
This compound serves as a building block in complex peptides like L-arginyl-L-alanyl-L-leucyl-L-α-aspartyl-L-phenylalanyl-alanine (CAS 574749-98-7). Such peptides are studied for their role in protein folding and drug design .
Research Findings and Implications
- NMR Spectroscopy : this compound’s isotropic NMR spectra (50–400 Hz linewidths) under magic-angle spinning (MAS) reveal its structural rigidity compared to flutamide or MDMA, which exhibit broader shifts due to aromatic interactions .
- Hydration Studies : Molecular dynamics simulations show that this compound’s carboxylate hydration closely mirrors glycine zwitterions, but its conformation is less sensitive to solvation than larger peptides like aspartylphenylalanine .
- Biological Relevance : While β-aspartylalanine is undetectable (>1 μM) in rat brains, its α-isomer may serve as a biomarker for peptide degradation or synthesis errors .
生物活性
Aspartylalanine, a dipeptide composed of aspartic acid and alanine, has garnered attention in various fields of biological research due to its unique properties and potential applications. This article explores its biological activity, mechanisms of action, and implications for therapeutic development.
Structure and Properties
This compound (CAS Number: 13433-02-8) is classified as a dipeptide, which consists of two amino acids linked by a peptide bond. Its molecular structure allows it to participate in various biochemical processes, particularly in the brain where it is involved in enhancing synaptic transmission . The compound's solubility and interaction with water molecules are critical for its function in biological systems, influencing its behavior within cellular environments.
Biological Functions
1. Role in Synaptic Transmission
This compound is naturally present in the brain and plays a significant role in enhancing synaptic transmission. Studies have shown that it increases the amplitude of miniature endplate potentials (MEPPs) in phrenic nerve-diaphragm preparations from rats, indicating its potential involvement in neurotransmission and neural signaling.
2. Interaction with Enzymes
This compound serves as a substrate for β-aspartyl peptidase, an enzyme that catalyzes the breakdown of peptides into their constituent amino acids. This enzymatic activity is crucial for various metabolic pathways, including the aspartate pathway, which is significant in synthesizing β-alanine .
3. Potential Therapeutic Applications
Research is ongoing to explore the therapeutic potential of this compound in neurological disorders. Its ability to enhance synaptic transmission suggests that it may have applications in treating conditions characterized by impaired neurotransmission. Furthermore, its properties as a model compound for peptide synthesis make it valuable in drug development and protein research.
The mechanisms through which this compound exerts its effects involve several biochemical pathways:
- Peptide Bond Formation : this compound can serve as a model substrate for studying peptide bond formation and cleavage by enzymes such as peptidases. Understanding these interactions aids in drug development and protein research.
- Hydrogen Bonding : The compound's ability to form hydrogen bonds allows it to self-organize into ordered structures, which can be utilized in designing self-assembling hydrogels for drug delivery applications.
- Metabolic Pathways : this compound participates in the metabolism of asparagine, an essential amino acid involved in nitrogen storage and transport within tissues. This metabolic role highlights its importance in maintaining cellular homeostasis .
Research Findings
Recent studies have provided insights into the biological activity of this compound:
-
Case Study: Metabolomic Profiling
A study on BALB/c macrophages infected with Leishmania demonstrated alterations in amino acid metabolism, including changes associated with L-arginine levels that could be influenced by dipeptides like this compound. The findings suggest that understanding these interactions may help identify new drug targets for controlling infections . -
Synthetic Applications
This compound has been explored for its potential use in synthetic peptides designed to mimic specific protein-protein interaction motifs crucial for cellular signaling. This application underscores its significance in developing novel therapeutic strategies.
Data Summary
Property | Value |
---|---|
Molecular Formula | C7H12N2O5 |
Molecular Weight | 188.18 g/mol |
Solubility | Highly soluble |
Biological Role | Enhances synaptic transmission |
Enzyme Interaction | β-aspartyl peptidase |
Future Directions
The ongoing research into this compound's biological activity suggests several promising avenues:
- Neuropharmacology : Further studies are needed to elucidate its precise role in neurotransmission and potential benefits in treating neurological disorders.
- Biomaterials Development : The properties of this compound may lead to innovative applications in creating biomaterials for drug delivery systems.
- Metabolic Studies : Investigating its interactions within metabolic pathways could provide insights into broader physiological processes and disease mechanisms.
Q & A
Basic Research Questions
Q. What analytical methods are commonly used to detect and quantify aspartylalanine in biological samples?
this compound is typically identified and quantified using high-performance liquid chromatography (HPLC) coupled with mass spectrometry (LC-MS/MS), especially in metabolomic studies. Sample preparation involves deproteinization (e.g., using acetonitrile) to isolate low-molecular-weight metabolites. Calibration curves with synthetic standards are essential for accurate quantification, and internal standards (e.g., isotopically labeled analogs) mitigate matrix effects .
Q. How is this compound synthesized for experimental use in laboratory settings?
Solid-phase peptide synthesis (SPPS) is a standard method for synthesizing this compound. The β-linked dipeptide requires protection of the aspartyl side chain (e.g., using tert-butyl esters) to prevent side reactions during coupling. Purification involves reversed-phase HPLC, with characterization via nuclear magnetic resonance (NMR) and high-resolution mass spectrometry (HRMS) to confirm purity and structure .
Q. What are the primary challenges in distinguishing this compound from structurally similar metabolites (e.g., β-aspartylglycine) during analysis?
Chromatographic separation using gradient elution (e.g., C18 columns with trifluoroacetic acid as an ion-pairing agent) improves resolution. Tandem mass spectrometry (MS/MS) with selective reaction monitoring (SRM) enhances specificity by targeting unique fragmentation patterns. Cross-validation with synthetic standards is critical to avoid misannotation .
Advanced Research Questions
Q. How can researchers address contradictions in reported concentrations of this compound across different studies (e.g., in rat cerebral cortex vs. human biofluids)?
Discrepancies may arise from methodological differences, such as sample preparation (e.g., extraction efficiency), detection limits of instruments, or interspecies variation. To resolve contradictions, studies should report detailed protocols for metabolite extraction, instrument parameters (e.g., LC gradient, MS ionization mode), and validation data (e.g., recovery rates, limits of detection). Meta-analyses using standardized workflows (e.g., METLIN database alignment) can harmonize data .
Q. What experimental designs are optimal for studying this compound’s role in hyperammonemia-related metabolic pathways?
Isotope tracing (e.g., ¹³C-labeled precursors) in cell or animal models can track this compound’s incorporation into nitrogen metabolism pathways. Pair this with knockdown/knockout models (e.g., CRISPR-Cas9 targeting glutamine synthetase) to assess functional roles. Multi-omics integration (metabolomics, proteomics) is recommended to contextualize its interactions with urea cycle enzymes .
Q. How should researchers validate the stability of this compound under varying physiological conditions (e.g., pH, temperature)?
Stability studies should simulate physiological extremes (e.g., pH 2–9, 4–37°C) and use accelerated degradation protocols. Monitor degradation products via LC-MS and calculate half-lives. Include stabilizing agents (e.g., antioxidants) in storage buffers if degradation exceeds acceptable thresholds (<15% loss over 24 hours) .
Q. What strategies improve the sensitivity of this compound detection in complex matrices like cerebrospinal fluid (CSF)?
Pre-concentration techniques, such as solid-phase extraction (SPE) with hydrophilic-lipophilic balance (HLB) cartridges, enhance sensitivity. Derivatization (e.g., dansyl chloride for fluorescence detection) or chemical isotope labeling (CIL) can amplify signals. Method validation must include spike-recovery experiments in CSF to confirm robustness against matrix interference .
Q. How do in vitro and in vivo quantification methods for this compound differ, and what biases might arise?
In vitro assays (e.g., enzyme kinetics) often use purified systems, avoiding metabolic degradation, whereas in vivo studies contend with rapid turnover and compartmentalization (e.g., blood-brain barrier transport). Correction factors for tissue-specific extraction efficiency and normalization to creatinine or protein content reduce biases .
Q. What computational tools are available to predict this compound’s interactions with enzymes like γ-glutamyl transpeptidase?
Molecular docking software (e.g., AutoDock Vina) and molecular dynamics simulations (e.g., GROMACS) model binding affinities and conformational changes. Pair these with kinetic assays (e.g., Michaelis-Menten parameters) to validate predictions. Structural data from crystallography (e.g., PDB entries) guide residue-specific mutagenesis studies .
Q. Tables for Methodological Reference
Table 1: Key Parameters for this compound Quantification via LC-MS/MS
Table 2: Common Artifacts in this compound Synthesis
Artifact | Cause | Mitigation Strategy |
---|---|---|
Racemization | High-temperature coupling | Use low-temperature (0–4°C) SPPS |
Side-chain oxidation | Deprotection under acidic conditions | Add scavengers (e.g., thioanisole) |
Truncated peptides | Incomplete coupling | Double coupling with HOBt/DIC |
特性
IUPAC Name |
(3S)-3-amino-4-[[(1S)-1-carboxyethyl]amino]-4-oxobutanoic acid | |
---|---|---|
Source | PubChem | |
URL | https://pubchem.ncbi.nlm.nih.gov | |
Description | Data deposited in or computed by PubChem | |
InChI |
InChI=1S/C7H12N2O5/c1-3(7(13)14)9-6(12)4(8)2-5(10)11/h3-4H,2,8H2,1H3,(H,9,12)(H,10,11)(H,13,14)/t3-,4-/m0/s1 | |
Source | PubChem | |
URL | https://pubchem.ncbi.nlm.nih.gov | |
Description | Data deposited in or computed by PubChem | |
InChI Key |
DVUFTQLHHHJEMK-IMJSIDKUSA-N | |
Source | PubChem | |
URL | https://pubchem.ncbi.nlm.nih.gov | |
Description | Data deposited in or computed by PubChem | |
Canonical SMILES |
CC(C(=O)O)NC(=O)C(CC(=O)O)N | |
Source | PubChem | |
URL | https://pubchem.ncbi.nlm.nih.gov | |
Description | Data deposited in or computed by PubChem | |
Isomeric SMILES |
C[C@@H](C(=O)O)NC(=O)[C@H](CC(=O)O)N | |
Source | PubChem | |
URL | https://pubchem.ncbi.nlm.nih.gov | |
Description | Data deposited in or computed by PubChem | |
Molecular Formula |
C7H12N2O5 | |
Source | PubChem | |
URL | https://pubchem.ncbi.nlm.nih.gov | |
Description | Data deposited in or computed by PubChem | |
DSSTOX Substance ID |
DTXSID10928569 | |
Record name | 3-Amino-4-[(1-carboxyethyl)imino]-4-hydroxybutanoic acid | |
Source | EPA DSSTox | |
URL | https://comptox.epa.gov/dashboard/DTXSID10928569 | |
Description | DSSTox provides a high quality public chemistry resource for supporting improved predictive toxicology. | |
Molecular Weight |
204.18 g/mol | |
Source | PubChem | |
URL | https://pubchem.ncbi.nlm.nih.gov | |
Description | Data deposited in or computed by PubChem | |
CAS No. |
13433-02-8 | |
Record name | Aspartylalanine | |
Source | CAS Common Chemistry | |
URL | https://commonchemistry.cas.org/detail?cas_rn=13433-02-8 | |
Description | CAS Common Chemistry is an open community resource for accessing chemical information. Nearly 500,000 chemical substances from CAS REGISTRY cover areas of community interest, including common and frequently regulated chemicals, and those relevant to high school and undergraduate chemistry classes. This chemical information, curated by our expert scientists, is provided in alignment with our mission as a division of the American Chemical Society. | |
Explanation | The data from CAS Common Chemistry is provided under a CC-BY-NC 4.0 license, unless otherwise stated. | |
Record name | alpha-Aspartylalanine | |
Source | ChemIDplus | |
URL | https://pubchem.ncbi.nlm.nih.gov/substance/?source=chemidplus&sourceid=0013433028 | |
Description | ChemIDplus is a free, web search system that provides access to the structure and nomenclature authority files used for the identification of chemical substances cited in National Library of Medicine (NLM) databases, including the TOXNET system. | |
Record name | 3-Amino-4-[(1-carboxyethyl)imino]-4-hydroxybutanoic acid | |
Source | EPA DSSTox | |
URL | https://comptox.epa.gov/dashboard/DTXSID10928569 | |
Description | DSSTox provides a high quality public chemistry resource for supporting improved predictive toxicology. | |
Retrosynthesis Analysis
AI-Powered Synthesis Planning: Our tool employs the Template_relevance Pistachio, Template_relevance Bkms_metabolic, Template_relevance Pistachio_ringbreaker, Template_relevance Reaxys, Template_relevance Reaxys_biocatalysis model, leveraging a vast database of chemical reactions to predict feasible synthetic routes.
One-Step Synthesis Focus: Specifically designed for one-step synthesis, it provides concise and direct routes for your target compounds, streamlining the synthesis process.
Accurate Predictions: Utilizing the extensive PISTACHIO, BKMS_METABOLIC, PISTACHIO_RINGBREAKER, REAXYS, REAXYS_BIOCATALYSIS database, our tool offers high-accuracy predictions, reflecting the latest in chemical research and data.
Strategy Settings
Precursor scoring | Relevance Heuristic |
---|---|
Min. plausibility | 0.01 |
Model | Template_relevance |
Template Set | Pistachio/Bkms_metabolic/Pistachio_ringbreaker/Reaxys/Reaxys_biocatalysis |
Top-N result to add to graph | 6 |
Feasible Synthetic Routes
試験管内研究製品の免責事項と情報
BenchChemで提示されるすべての記事および製品情報は、情報提供を目的としています。BenchChemで購入可能な製品は、生体外研究のために特別に設計されています。生体外研究は、ラテン語の "in glass" に由来し、生物体の外で行われる実験を指します。これらの製品は医薬品または薬として分類されておらず、FDAから任何の医療状態、病気、または疾患の予防、治療、または治癒のために承認されていません。これらの製品を人間または動物に体内に導入する形態は、法律により厳格に禁止されています。これらのガイドラインに従うことは、研究と実験において法的および倫理的な基準の遵守を確実にするために重要です。