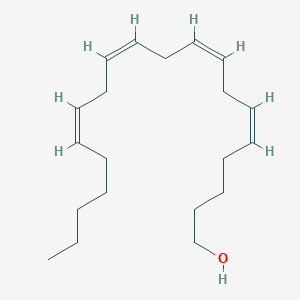
Arachidonyl alcohol
概要
説明
Arachidonyl alcohol (C₂₀H₃₂O, IUPAC name: 5Z,8Z,11Z,14Z-eicosatetraen-1-ol) is a long-chain primary fatty alcohol characterized by a 20-carbon chain with four cis double bonds at positions 5, 8, 11, and 14 . It is structurally derived from arachidonic acid (AA), differing by the replacement of the carboxylic acid (-COOH) group with a hydroxyl (-OH) group. This compound is primarily utilized as a substrate for synthesizing ether lipids, which are critical components of cellular membranes and signaling molecules . Its physicochemical properties, such as hydrophobicity and membrane permeability, are influenced by its unsaturated structure, enabling interactions with lipid-binding proteins and enzymes .
準備方法
Chemical Reduction of Arachidonic Acid
The reduction of arachidonic acid (5Z,8Z,11Z,14Z-eicosatetraenoic acid) to arachidonyl alcohol represents the most direct and widely employed synthetic route. This method leverages strong reducing agents to convert the carboxylic acid group into a primary alcohol.
Lithium Aluminum Hydride (LiAlH4_44) Reduction
Lithium aluminum hydride is a classical reducing agent for converting carboxylic acids to primary alcohols. In the case of arachidonic acid, the reaction proceeds via a two-step mechanism:
-
Formation of the carboxylate intermediate :
-
Reduction to the primary alcohol :
Reaction Conditions :
-
Solvent : Anhydrous diethyl ether or tetrahydrofuran (THF)
-
Temperature : 0°C to room temperature, under inert atmosphere
-
Workup : Sequential quenching with wet ether, aqueous HCl, and extraction with organic solvents
Yield : Typical yields range from 70% to 85%, contingent on the purity of arachidonic acid and exclusion of moisture .
Catalytic Hydrogenation
Catalytic hydrogenation offers a milder alternative, employing transition metal catalysts to reduce the carboxylic acid group. Palladium-based catalysts, such as Pd/BaSO, are preferred due to their selectivity and tolerance for polyunsaturated bonds.
Reaction Scheme :
2 \xrightarrow{\text{Pd/BaSO}4} \text{RCH}_2\text{OH}
Optimized Parameters :
-
Pressure : 1–3 atm H
-
Temperature : 25–40°C
-
Solvent : Ethanol or ethyl acetate
Advantages :
-
Reduced risk of over-reduction compared to LiAlH
Yield : Reported yields exceed 90% under optimized conditions .
Microbial Biosynthesis Using Acinetobacter Species
Microbial conversion provides a sustainable and stereoselective route to this compound. Toshihiro Nagao and colleagues demonstrated the efficacy of Acinetobacter sp. strain KY-1 in catalyzing this transformation .
Fermentation Protocol
-
Culture Conditions :
-
Medium : Soybean oil (20 g/L) as carbon source, peptone (10 g/L), yeast extract (5 g/L)
-
Temperature : 30°C, aerobic agitation (200 rpm)
-
Incubation Time : 72–96 hours
-
-
Enzymatic Mechanism :
-
Acyl-CoA synthase activates arachidonic acid to arachidonoyl-CoA.
-
Fatty alcohol reductase catalyzes the NADPH-dependent reduction of arachidonoyl-CoA to this compound.
-
Yield : The strain KY-1 produces this compound at 0.8–1.2 g/L, with >95% stereochemical purity .
Advantages Over Chemical Methods
-
Stereoselectivity : Exclusive formation of (5Z,8Z,11Z,14Z) isomer.
-
Sustainability : Utilizes renewable substrates (e.g., soybean oil) and aqueous reaction media .
Comparative Analysis of Synthesis Methods
The choice of method hinges on scalability, cost, and desired purity. The table below summarizes key metrics:
Method | Yield (%) | Purity (%) | Cost (Relative) | Scalability |
---|---|---|---|---|
LiAlH Reduction | 70–85 | 85–90 | Low | Laboratory-scale |
Catalytic Hydrogenation | >90 | 90–95 | Moderate | Pilot-scale |
Microbial Biosynthesis | 80–95 | >95 | High | Industrial-scale |
Key Observations :
化学反応の分析
反応の種類
アラキドノイルアルコールは、以下を含むさまざまな化学反応を起こします。
酸化: アラキドノイルアルコールは酸化されて対応するアルデヒドとカルボン酸を形成することができます。
還元: この化合物はさらに還元されて飽和アルコールを形成することができます。
置換: アラキドノイルアルコールは、ヒドロキシル基が他の官能基に置き換わる置換反応に関与することができます。
一般的な試薬と条件
酸化: 一般的な酸化剤には、過マンガン酸カリウム(KMnO4)と三酸化クロム(CrO3)が含まれます。
還元: 水素化リチウムアルミニウム(LiAlH4)は、還元反応に一般的に使用されます。
置換: 塩化チオニル(SOCl2)などの試薬は、ヒドロキシル基を塩素原子に置き換えるために使用できます。
生成される主な製品
酸化: アラキドン酸および他のカルボン酸。
還元: 飽和脂肪アルコール。
置換: 使用される試薬に応じて、さまざまな置換誘導体。
科学研究の用途
アラキドノイルアルコールは、科学研究で幅広い用途があります。
化学: これは、有益な機能を持つさまざまなエーテル脂質の合成の基質として使用されます。
生物学: アラキドノイルアルコールは、脂質代謝とシグナル伝達経路の研究に関与しています。
医学: アラキドノイルエタノールアミドなどのアラキドノイルアルコールの誘導体は、カンナビノイド受容体の内因性リガンドであり、潜在的な医療上の利点があります.
科学的研究の応用
Arachidonyl alcohol has a wide range of applications in scientific research:
Chemistry: It is used as a substrate for the synthesis of various ether lipids, which have beneficial functions.
Biology: this compound is involved in the study of lipid metabolism and signaling pathways.
作用機序
アラキドノイルアルコールは、さまざまな分子標的と経路を通じてその効果を発揮します。これは、プロスタグランジン、ロイコトリエン、トロンボキサンなど、いくつかの生物活性脂質の前駆体であるアラキドン酸の代謝に関与しています。 これらの脂質は、炎症、免疫応答、その他の生理学的プロセスで重要な役割を果たしています .
類似の化合物との比較
アラキドノイルアルコールは、以下のような他の類似の化合物と比較することができます。
アナンダミド(アラキドノイルエタノールアミド): どちらもアラキドン酸から誘導され、カンナビノイド受容体のリガンドとして機能します.
2-アラキドノイルグリセロール: カンナビノイド受容体と相互作用する別のエンドカンナビノイド.
アラキドン酸: アラキドノイルアルコールおよび他の生物活性脂質の前駆体.
アラキドノイルアルコールは、その特定の構造と複数のシス二重結合の存在により、独自の化学的および生物学的特性を持っています。
類似化合物との比較
Comparison with Structurally Similar Compounds
Arachidonic Acid (AA) and Derivatives
Key Findings :
- Functional Group Impact: The hydroxyl group in this compound renders it ineffective in pathways requiring the carboxylic acid group of AA, such as eicosanoid synthesis or prostaglandin-mediated gene expression .
- G-Protein Modulation : Both this compound and AA inhibit GTPγS binding to Gzα, suggesting their unsaturated chains disrupt G-protein interactions. However, AA’s carboxylic acid group enhances this activity .
- Receptor Selectivity: Unlike AA derivatives like anandamide or MAFP, this compound lacks affinity for cannabinoid receptors, highlighting the necessity of specific functional groups (e.g., amide in AEA, fluorophosphonate in MAFP) for receptor binding .
Other Unsaturated Fatty Alcohols and Acids
Key Findings :
- Chain Length and Unsaturation : this compound’s tetraunsaturated 20-carbon chain is critical for its inhibitory effects on GTPγS binding, as saturated analogs (e.g., arachidic acid) are inactive .
- Enzyme Specificity : Unlike OA, which enhances PKA activity, this compound shows negligible effects on cAMP-dependent PKA, emphasizing the role of both chain structure and functional groups .
生物活性
Arachidonyl alcohol (AA), a fatty alcohol derived from arachidonic acid, has garnered attention in the field of pharmacology and neuroscience due to its interactions with the endocannabinoid system (ECS) and its potential implications in various biological processes. This article explores the biological activity of this compound, focusing on its mechanisms of action, effects on neurotransmission, and implications for addiction and neuroprotection.
Chemical Structure and Properties
This compound is an unsaturated fatty alcohol with the following chemical structure:
- Chemical Formula: C20H36O
- Molecular Weight: 304.5 g/mol
This compound is structurally related to arachidonic acid, a key precursor for various signaling molecules, including endocannabinoids.
This compound interacts primarily with the cannabinoid receptors (CB1 and CB2), which are part of the ECS. The ECS plays a crucial role in modulating neurotransmission, pain perception, and various physiological processes.
Interaction with Cannabinoid Receptors
- CB1 Receptor Activation: this compound acts as a partial agonist at the CB1 receptor, influencing neurotransmitter release and neuronal excitability. Studies have shown that it can induce rapid transient increases in intracellular calcium levels in neuronal cells through a CB1-dependent mechanism .
- CB2 Receptor Modulation: While less studied, there is evidence suggesting that this compound may also interact with CB2 receptors, which are primarily involved in immune responses.
Neurotransmission
Research indicates that this compound modulates glutamatergic transmission. Ethanol exposure has been shown to alter the levels of endocannabinoids like anandamide (AEA) and 2-arachidonoylglycerol (2-AG), which are crucial for synaptic plasticity . this compound may influence these pathways by affecting the synthesis or degradation of these endocannabinoids.
Alcohol Interaction
Studies have demonstrated that chronic alcohol exposure impacts the ECS by increasing AEA levels while decreasing 2-AG levels in various brain regions . This suggests that this compound may play a role in mediating some effects of alcohol on the brain, potentially influencing behaviors associated with addiction.
Study 1: Ethanol and Endocannabinoid Levels
A study conducted on male Wistar rats showed that chronic ethanol exposure resulted in significant alterations in endocannabinoid signaling. Specifically, it was observed that chronic exposure increased AEA content while withdrawal led to decreased levels . This fluctuation may be mediated by this compound's effects on cannabinoid receptor signaling.
Study 2: Behavioral Responses
Research involving FAAH (fatty acid amide hydrolase) knockout mice illustrated that elevated levels of AEA due to inhibited degradation resulted in increased alcohol self-administration behaviors . This underscores the importance of this compound and related compounds in addiction pathways.
Data Summary Table
Study | Findings | Implications |
---|---|---|
Pava et al. (2012) | Chronic ethanol alters ECS components, affecting CB1 expression | Suggests role of this compound in addiction |
Naassila et al. (2004) | Mice lacking CB1 receptors show reduced ethanol self-administration | Highlights importance of ECS in alcohol behavior |
Cravatt et al. (1996) | FAAH inhibition leads to increased AEA levels | Indicates potential therapeutic targets |
Q & A
Basic Research Questions
Q. What are the established methods for synthesizing arachidonyl alcohol in laboratory settings?
this compound (C20H36O) is typically synthesized via esterification or reduction of arachidonic acid derivatives. A common approach involves catalytic hydrogenation of arachidonyl esters (e.g., methyl arachidonate) using palladium-based catalysts under controlled hydrogen pressure. Purity validation requires gas chromatography with flame ionization detection (GC-FID) or nuclear magnetic resonance (NMR) spectroscopy to confirm structural integrity and absence of unsaturated byproducts .
Q. Which analytical techniques are recommended for structural characterization of this compound?
- NMR Spectroscopy : Proton (¹H) and carbon-13 (¹³C) NMR are critical for identifying functional groups and carbon-chain conformation.
- Mass Spectrometry (MS) : High-resolution MS (HRMS) confirms molecular weight (284.5 g/mol) and fragmentation patterns.
- GC-FID : Quantifies purity and detects impurities in synthetic batches .
Q. How can researchers ensure accurate quantification of this compound in biological matrices?
Use dual analytical methods (e.g., GC-FID paired with enzymatic assays) to cross-validate results. Pre-analytical steps must include sample stabilization with antioxidants (e.g., BHT) to prevent oxidation and storage at -80°C to preserve integrity. Report results as the average of ≥2 replicates, rounded to two decimal places to minimize measurement bias .
Advanced Research Questions
Q. What experimental strategies address contradictions in reported bioactivity of this compound across studies?
Discrepancies may arise from differences in model systems (e.g., in vitro vs. in vivo) or lipid matrix interactions. To resolve this:
- Control for confounding factors : Standardize lipid extraction protocols (e.g., Folch method) and account for interspecies variations in lipid metabolism.
- Dose-response studies : Use isothermal titration calorimetry (ITC) to quantify binding affinities in lipid bilayers .
Q. How does this compound modulate lipid signaling pathways, and what methodologies elucidate its mechanistic role?
this compound serves as a substrate for ether lipid biosynthesis, influencing prostaglandin and endocannabinoid pathways. Methodologies include:
- Isotopic labeling : Track incorporation into phosphatidylethanolamine derivatives using ¹⁴C-labeled this compound.
- Knockout models : CRISPR/Cas9-mediated deletion of AGPS (alkylglycerone phosphate synthase) to study ether lipid deficiency .
Q. What are the challenges in isolating this compound from complex biological samples, and how can they be mitigated?
Challenges include low endogenous concentrations and co-elution with structurally similar lipids. Solutions involve:
- Solid-phase extraction (SPE) : Use C18 columns with methanol/chloroform gradients.
- Derivatization : Convert to pentafluorobenzoyl esters for enhanced GC-MS sensitivity .
Q. Methodological Best Practices
Q. How should researchers design experiments to study the oxidation stability of this compound?
- Accelerated stability testing : Expose samples to 40°C/75% RH and monitor degradation via thin-layer chromatography (TLC).
- Antioxidant screening : Compare efficacy of α-tocopherol vs. ascorbyl palmitate in lipid matrices .
Q. What statistical approaches are optimal for analyzing dose-dependent effects of this compound in cell cultures?
Use nonlinear regression models (e.g., sigmoidal dose-response curves) in software like GraphPad Prism. Report EC₅₀/IC₅₀ values with 95% confidence intervals and validate assumptions via Kolmogorov-Smirnov normality tests .
Q. Data Reporting and Validation
Q. How should conflicting data on this compound’s receptor interactions be resolved?
- Competitive binding assays : Compare displacement of radiolabeled ligands (e.g., [³H]anandamide) in receptor-rich membranes.
- Molecular docking simulations : Predict binding affinities using AutoDock Vina and validate with mutagenesis studies .
Q. What metadata are critical to include when publishing this compound research?
- Pre-analytical details : Sampling time, storage conditions, and anticoagulants used.
- Instrument parameters : GC oven temperature, MS ionization modes.
- Uncertainty estimates : Report ±SD or confidence intervals for quantitative assays .
Q. Comparative Table: Analytical Methods for this compound
Method | Sensitivity (LOD) | Key Application | Limitations |
---|---|---|---|
GC-FID | 0.1 µg/mL | Purity assessment | Limited structural detail |
NMR | 1.0 µg/mL | Structural elucidation | High sample requirement |
HRMS | 0.01 µg/mL | Molecular weight confirmation | Cost-intensive |
LC-MS/MS | 0.05 µg/mL | Trace quantification in biomatrices | Matrix interference risks |
特性
IUPAC Name |
(5Z,8Z,11Z,14Z)-icosa-5,8,11,14-tetraen-1-ol | |
---|---|---|
Source | PubChem | |
URL | https://pubchem.ncbi.nlm.nih.gov | |
Description | Data deposited in or computed by PubChem | |
InChI |
InChI=1S/C20H34O/c1-2-3-4-5-6-7-8-9-10-11-12-13-14-15-16-17-18-19-20-21/h6-7,9-10,12-13,15-16,21H,2-5,8,11,14,17-20H2,1H3/b7-6-,10-9-,13-12-,16-15- | |
Source | PubChem | |
URL | https://pubchem.ncbi.nlm.nih.gov | |
Description | Data deposited in or computed by PubChem | |
InChI Key |
NYBCZSBDKXGAGM-DOFZRALJSA-N | |
Source | PubChem | |
URL | https://pubchem.ncbi.nlm.nih.gov | |
Description | Data deposited in or computed by PubChem | |
Canonical SMILES |
CCCCCC=CCC=CCC=CCC=CCCCCO | |
Source | PubChem | |
URL | https://pubchem.ncbi.nlm.nih.gov | |
Description | Data deposited in or computed by PubChem | |
Isomeric SMILES |
CCCCC/C=C\C/C=C\C/C=C\C/C=C\CCCCO | |
Source | PubChem | |
URL | https://pubchem.ncbi.nlm.nih.gov | |
Description | Data deposited in or computed by PubChem | |
Molecular Formula |
C20H34O | |
Source | PubChem | |
URL | https://pubchem.ncbi.nlm.nih.gov | |
Description | Data deposited in or computed by PubChem | |
DSSTOX Substance ID |
DTXSID30447409 | |
Record name | Arachidonyl alcohol | |
Source | EPA DSSTox | |
URL | https://comptox.epa.gov/dashboard/DTXSID30447409 | |
Description | DSSTox provides a high quality public chemistry resource for supporting improved predictive toxicology. | |
Molecular Weight |
290.5 g/mol | |
Source | PubChem | |
URL | https://pubchem.ncbi.nlm.nih.gov | |
Description | Data deposited in or computed by PubChem | |
CAS No. |
13487-46-2 | |
Record name | Arachidonyl alcohol | |
Source | CAS Common Chemistry | |
URL | https://commonchemistry.cas.org/detail?cas_rn=13487-46-2 | |
Description | CAS Common Chemistry is an open community resource for accessing chemical information. Nearly 500,000 chemical substances from CAS REGISTRY cover areas of community interest, including common and frequently regulated chemicals, and those relevant to high school and undergraduate chemistry classes. This chemical information, curated by our expert scientists, is provided in alignment with our mission as a division of the American Chemical Society. | |
Explanation | The data from CAS Common Chemistry is provided under a CC-BY-NC 4.0 license, unless otherwise stated. | |
Record name | Arachidonyl alcohol | |
Source | EPA DSSTox | |
URL | https://comptox.epa.gov/dashboard/DTXSID30447409 | |
Description | DSSTox provides a high quality public chemistry resource for supporting improved predictive toxicology. | |
Retrosynthesis Analysis
AI-Powered Synthesis Planning: Our tool employs the Template_relevance Pistachio, Template_relevance Bkms_metabolic, Template_relevance Pistachio_ringbreaker, Template_relevance Reaxys, Template_relevance Reaxys_biocatalysis model, leveraging a vast database of chemical reactions to predict feasible synthetic routes.
One-Step Synthesis Focus: Specifically designed for one-step synthesis, it provides concise and direct routes for your target compounds, streamlining the synthesis process.
Accurate Predictions: Utilizing the extensive PISTACHIO, BKMS_METABOLIC, PISTACHIO_RINGBREAKER, REAXYS, REAXYS_BIOCATALYSIS database, our tool offers high-accuracy predictions, reflecting the latest in chemical research and data.
Strategy Settings
Precursor scoring | Relevance Heuristic |
---|---|
Min. plausibility | 0.01 |
Model | Template_relevance |
Template Set | Pistachio/Bkms_metabolic/Pistachio_ringbreaker/Reaxys/Reaxys_biocatalysis |
Top-N result to add to graph | 6 |
Feasible Synthetic Routes
試験管内研究製品の免責事項と情報
BenchChemで提示されるすべての記事および製品情報は、情報提供を目的としています。BenchChemで購入可能な製品は、生体外研究のために特別に設計されています。生体外研究は、ラテン語の "in glass" に由来し、生物体の外で行われる実験を指します。これらの製品は医薬品または薬として分類されておらず、FDAから任何の医療状態、病気、または疾患の予防、治療、または治癒のために承認されていません。これらの製品を人間または動物に体内に導入する形態は、法律により厳格に禁止されています。これらのガイドラインに従うことは、研究と実験において法的および倫理的な基準の遵守を確実にするために重要です。