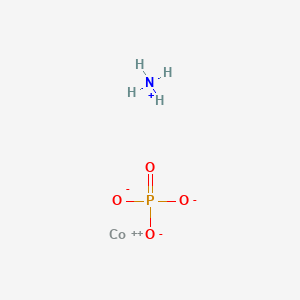
リン酸コバルトアンモニウム
概要
説明
Ammonium cobalt phosphate is an inorganic compound with the chemical formula ( \text{NH}_4\text{CoPO}_4 ). It is a member of the cobalt phosphate family, which is known for its diverse applications in various fields such as catalysis, energy storage, and materials science. The compound is characterized by its unique crystalline structure and the presence of cobalt in its oxidation state, which imparts distinct chemical and physical properties.
科学的研究の応用
Electrocatalysis
Ammonium cobalt phosphate has emerged as a promising electrocatalyst for several electrochemical reactions, particularly the oxygen evolution reaction (OER) and nitrogen reduction reaction (NRR).
Oxygen Evolution Reaction
Recent studies highlight the effectiveness of ammonium cobalt phosphate nanosheets as electrocatalysts for alkaline saline water oxidation. The nanosheet structure enhances the active surface area, leading to improved catalytic performance.
- Performance Metrics :
- Current densities of 10 mA cm⁻² and 100 mA cm⁻² achieved at overpotentials of 252 mV and 268 mV, respectively.
- Remarkable stability during operation at room temperature.
This performance positions ammonium cobalt phosphate as a cost-effective alternative to noble metals in water electrolysis technology aimed at clean hydrogen production .
Nitrogen Reduction Reaction
Ammonium cobalt phosphate has also been utilized in electrochemical nitrogen reduction processes. Hollow cobalt phosphate microspheres derived from ammonium cobalt phosphate serve as efficient electrocatalysts, achieving high ammonia yield rates with significant faradaic efficiency under alkaline conditions.
- Key Findings :
- Ammonia yield rate: 16.48 μg h⁻¹ mg cat.⁻¹.
- Faradaic efficiency: 4.46% at -0.2 V vs. reversible hydrogen electrode.
These findings suggest that ammonium cobalt phosphate can play a crucial role in sustainable ammonia production through electrochemical methods .
Energy Storage
The unique structural characteristics of ammonium cobalt phosphate make it suitable for applications in supercapacitors, which are vital for energy storage systems.
Supercapacitor Applications
Research has demonstrated that two-dimensional lamellar structures of ammonium cobalt phosphate significantly enhance energy storage capabilities.
- Performance Metrics :
- Specific capacitance: Up to 585.83 F·g⁻¹ at a current density of 0.6 A·g⁻¹.
- Retention of specific capacitance: Maintained at 87.13% after 4000 charge-discharge cycles at a current density of 10 A·g⁻¹.
These results indicate that ammonium cobalt phosphate materials can be effectively utilized in high-performance supercapacitors, contributing to advancements in energy storage technologies .
Waste Management and Recycling
Ammonium cobalt phosphate is also relevant in the context of industrial waste management, particularly in recycling processes for cobalt-containing waste solutions.
Cobalt Recovery from Industrial Waste
A patented method utilizes spent solutions from galvanic and chemical coatings to synthesize ammonium cobalt phosphates, thereby recovering valuable cobalt while minimizing environmental impact.
- Process Overview :
- Spent solutions containing cobalt (II) are treated with non-toxic reagents to precipitate ammonium cobalt phosphates.
- This method not only recycles cobalt but also produces high-value chemical compounds suitable for industrial and agricultural applications.
This approach underscores the potential for ammonium cobalt phosphate in sustainable practices within the chemical industry .
作用機序
Target of Action
Ammonium cobalt phosphate (also known as Ammonium cobaltous phosphate) primarily targets the oxygen evolution reaction (OER) and nitrogen reduction reaction (NRR) in the field of electrocatalysis . These reactions are crucial for advancing water splitting technology to generate clean hydrogen energy .
Mode of Action
Ammonium cobalt phosphate interacts with its targets through a series of electrochemical processes. The activation of Co(II) ions in NH4CoPO4·H2O to Co(III) species constructs the electrocatalytic active sites . The Co 4p orbitals directly participate in the nitrate adsorption and electron transfer step of the NO3− RR .
Biochemical Pathways
The compound plays a significant role in the electrochemical nitrogen reduction to produce ammonia, a process that overcomes the drawbacks of the energy-intensive Haber–Bosch reaction and low-efficient N2 electroreduction . Phosphorus is crucial for stabilizing the active phase and optimizing the energy barriers of the NO3− RR .
Pharmacokinetics
The compound exhibits remarkable stability during the alkaline saline water oxidation at room temperature .
Result of Action
The compound exhibits high electrocatalytic activity. When used for alkaline saline water electrolysis, the NH4CoPO4·H2O catalyst achieves current densities of 10 and 100 mA cm−2 at the record low overpotentials of 252 and 268 mV, respectively . The 2D nanosheet morphology of NH4CoPO4·H2O provides a larger active surface area and more surface-exposed active sites .
Action Environment
The action, efficacy, and stability of ammonium cobalt phosphate are influenced by environmental factors such as temperature and pH. The compound maintains remarkable stability during the alkaline saline water oxidation at room temperature . The compound’s action is also influenced by the Co/Ni molar ratios, which can significantly influence the crystal structure and morphologies of NH4CoPO4·H2O .
生化学分析
Cellular Effects
Cobalt-based materials, including cobalt phosphates, have been shown to have significant electrocatalytic activity . This suggests that ammonium cobalt phosphate could potentially influence cellular functions, including cell signaling pathways, gene expression, and cellular metabolism.
Molecular Mechanism
It is known that cobalt phosphates can form various physical structures, which may influence their biochemical and cellular effects
準備方法
Synthetic Routes and Reaction Conditions: Ammonium cobalt phosphate can be synthesized through various methods, including hydrothermal synthesis and chemical precipitation. One common method involves the reaction of cobalt nitrate with ammonium dihydrogen phosphate in an aqueous solution. The reaction is typically carried out under controlled temperature and pH conditions to ensure the formation of the desired crystalline phase.
Industrial Production Methods: In industrial settings, the production of ammonium cobalt phosphate often involves large-scale hydrothermal synthesis. This method allows for the precise control of reaction parameters, leading to high-purity products. The process typically involves the use of autoclaves to maintain high pressure and temperature, facilitating the formation of the crystalline structure.
化学反応の分析
Types of Reactions: Ammonium cobalt phosphate undergoes various chemical reactions, including:
Oxidation: The cobalt ions in the compound can be oxidized, leading to changes in the oxidation state and the formation of different cobalt oxides.
Reduction: The compound can also undergo reduction reactions, where the cobalt ions are reduced to lower oxidation states.
Substitution: Ammonium ions in the compound can be substituted with other cations, leading to the formation of different cobalt phosphate derivatives.
Common Reagents and Conditions:
Oxidation: Common oxidizing agents include hydrogen peroxide and potassium permanganate.
Reduction: Reducing agents such as sodium borohydride and hydrazine are often used.
Substitution: Various metal salts can be used to introduce different cations into the structure.
Major Products Formed:
Oxidation: Cobalt oxides such as cobalt(III) oxide.
Reduction: Lower oxidation state cobalt compounds.
Substitution: Different metal phosphates depending on the substituting cation.
類似化合物との比較
Ammonium cobalt phosphate can be compared with other similar compounds, such as:
Cobalt phosphate: Similar in structure but lacks the ammonium ion, leading to different chemical properties.
Nickel phosphate: Similar in structure but contains nickel instead of cobalt, resulting in different electrochemical properties.
Iron phosphate: Contains iron instead of cobalt, leading to different magnetic and catalytic properties.
Uniqueness: Ammonium cobalt phosphate is unique due to the presence of both ammonium and cobalt ions, which impart distinct chemical and physical properties. Its ability to undergo various redox reactions and its excellent electrochemical performance make it a valuable compound in both research and industrial applications.
生物活性
Ammonium cobalt phosphate (NH₄CoPO₄) is a compound that has garnered interest in various fields, particularly due to its biological activity and potential applications in medicine and environmental science. This article explores the biological activity of ammonium cobalt phosphate, including its physiological effects, potential therapeutic uses, and relevant research findings.
Ammonium cobalt phosphate is an inorganic compound characterized by its blue crystalline structure. It is classified under cobalt phosphate salts, with the following chemical properties:
- Molecular Formula : NH₄CoPO₄
- CAS Number : 14590-13-7
- Appearance : Blue powder
- Solubility : Sparingly soluble in water
Toxicological Studies
Research indicates that ammonium cobalt phosphate exhibits both beneficial and harmful biological effects. A tier II human health assessment evaluated cobalt salts, including ammonium cobalt phosphate, revealing several key findings:
- Acute Toxicity : The compound has been shown to cause sub-lethal effects such as ataxia, tremor, and dyspnea at concentrations as low as 1.07 mg/L. The calculated LC50 (lethal concentration for 50% of the population) was determined to be approximately 0.06 mg/L, indicating significant toxicity at elevated levels .
- Skin Irritation : In vitro studies have demonstrated that ammonium cobalt phosphate does not cause skin irritation or corrosivity in human-derived epidermal keratinocytes, suggesting a relatively low risk for dermal exposure .
Therapeutic Applications
Despite its toxicity at high concentrations, cobalt compounds have been explored for their therapeutic potential:
- Anemia Treatment : Cobalt salts have historically been used to treat anemia due to their ability to stimulate erythropoiesis (the production of red blood cells). Cobalt therapy has been shown to increase hemoglobin levels in patients through mechanisms that are still being researched .
- Potential for Cancer Treatment : Some studies suggest that cobalt compounds may induce apoptosis (programmed cell death) in cancer cells. This effect could be attributed to oxidative stress mechanisms triggered by cobalt ions .
Environmental Impact
Ammonium cobalt phosphate also plays a role in environmental biology:
- Microbial Interactions : Research has demonstrated the use of cobalt-based microelectrodes for monitoring phosphate levels in microbial flocs. These microelectrodes exhibit excellent selectivity for orthophosphate ions and can provide valuable data for understanding microbial processes in wastewater treatment .
Case Study 1: Erythropoiesis Stimulation
A clinical study investigated the effects of cobalt chloride on patients with chronic kidney disease. Patients received daily doses ranging from 0.65 to 4.0 mg/kg/day over several weeks. The results indicated a significant increase in erythrocyte counts and hemoglobin levels, reducing the need for blood transfusions .
Case Study 2: Environmental Monitoring
In a study focused on wastewater treatment, cobalt-based microelectrodes were employed to monitor phosphate levels within microbial flocs under anaerobic conditions. The findings revealed distinct phosphate profiles that correlated with microbial activity, underscoring the importance of these electrodes in environmental monitoring .
Research Findings Summary Table
特性
CAS番号 |
14590-13-7 |
---|---|
分子式 |
CoH6NO4P |
分子量 |
173.96 g/mol |
IUPAC名 |
azane;cobalt;phosphoric acid |
InChI |
InChI=1S/Co.H3N.H3O4P/c;;1-5(2,3)4/h;1H3;(H3,1,2,3,4) |
InChIキー |
ASYZRLCMYUFCHK-UHFFFAOYSA-N |
SMILES |
[NH4+].[O-]P(=O)([O-])[O-].[Co+2] |
正規SMILES |
N.OP(=O)(O)O.[Co] |
Key on ui other cas no. |
36835-61-7 14590-13-7 |
ピクトグラム |
Irritant |
関連するCAS |
36835-61-7 |
製品の起源 |
United States |
Retrosynthesis Analysis
AI-Powered Synthesis Planning: Our tool employs the Template_relevance Pistachio, Template_relevance Bkms_metabolic, Template_relevance Pistachio_ringbreaker, Template_relevance Reaxys, Template_relevance Reaxys_biocatalysis model, leveraging a vast database of chemical reactions to predict feasible synthetic routes.
One-Step Synthesis Focus: Specifically designed for one-step synthesis, it provides concise and direct routes for your target compounds, streamlining the synthesis process.
Accurate Predictions: Utilizing the extensive PISTACHIO, BKMS_METABOLIC, PISTACHIO_RINGBREAKER, REAXYS, REAXYS_BIOCATALYSIS database, our tool offers high-accuracy predictions, reflecting the latest in chemical research and data.
Strategy Settings
Precursor scoring | Relevance Heuristic |
---|---|
Min. plausibility | 0.01 |
Model | Template_relevance |
Template Set | Pistachio/Bkms_metabolic/Pistachio_ringbreaker/Reaxys/Reaxys_biocatalysis |
Top-N result to add to graph | 6 |
Feasible Synthetic Routes
試験管内研究製品の免責事項と情報
BenchChemで提示されるすべての記事および製品情報は、情報提供を目的としています。BenchChemで購入可能な製品は、生体外研究のために特別に設計されています。生体外研究は、ラテン語の "in glass" に由来し、生物体の外で行われる実験を指します。これらの製品は医薬品または薬として分類されておらず、FDAから任何の医療状態、病気、または疾患の予防、治療、または治癒のために承認されていません。これらの製品を人間または動物に体内に導入する形態は、法律により厳格に禁止されています。これらのガイドラインに従うことは、研究と実験において法的および倫理的な基準の遵守を確実にするために重要です。