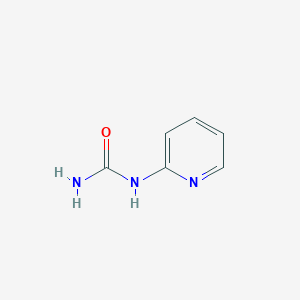
ピリジン-2-イル-尿素
概要
説明
Pyridin-2-yl-urea is an organic compound that features a urea moiety attached to a pyridine ring. This compound is of significant interest due to its versatile applications in medicinal chemistry and materials science. The presence of the pyridine ring imparts unique chemical and biological properties, making it a valuable scaffold in drug design and other scientific research.
科学的研究の応用
Medicinal Chemistry Applications
Anticancer Activity
Pyridin-2-yl-urea derivatives have shown promising results in anticancer research. Several studies highlight their effectiveness against various cancer cell lines:
- VEGFR2 Inhibition : Pyridine–urea compounds are known for their role as inhibitors of the vascular endothelial growth factor receptor 2 (VEGFR2), which is crucial in tumor angiogenesis. A study demonstrated that specific pyridin-2-yl urea compounds exhibited submicromolar activity against breast (MCF7) and colon (HCT116) cancer cell lines, with one compound achieving a GI50 value of 0.06 μM against MCF7 cells .
- Copper Complexes : Recent research synthesized novel Cu(II) complexes from pyridin-2-yl urea derivatives, revealing significant antiproliferative effects, particularly against drug-resistant lung cancer cell lines. The study noted that certain complexes showed IC50 values as low as 33.4 μM, indicating their potential as effective anticancer agents .
Synthesis and Reactivity
Synthesis Protocols
The synthesis of pyridin-2-yl urea can be achieved through various methods, including:
- Ammonolysis Reactions : A metal-free one-pot ammonolysis approach has been developed to synthesize unsymmetrical ureas bearing 2-pyridyl units, resulting in nearly quantitative yields . This method is advantageous due to its simplicity and scalability.
- Hybridization Strategies : The integration of hybridization strategies has led to the creation of novel small molecules combining urea and pyridine structures. These compounds have been tested for their anticancer properties, showcasing the versatility of pyridin-2-yl urea derivatives in drug design .
Material Science Applications
Coordination Chemistry
Pyridin-2-yl urea derivatives have also been explored in coordination chemistry:
- Metal Complex Formation : The ability of pyridin-2-yl urea to form stable complexes with transition metals like copper has been investigated. These complexes not only exhibit unique structural properties but also display significant biological activities, making them potential candidates for further development in therapeutic applications .
Case Studies
作用機序
Target of Action
Pyridin-2-yl-urea has been identified as a potential inhibitor of ASK1 . ASK1 (Apoptosis Signal-regulating Kinase 1) is a member of the MAP kinase family, which plays a critical role in cellular responses to stress, such as oxidative stress, endoplasmic reticulum stress, and calcium influx .
Mode of Action
It is speculated that the compound may interact with ask1, leading to its inhibition . This inhibition could potentially prevent the activation of downstream signaling pathways that are involved in stress responses and apoptosis .
Biochemical Pathways
Upon inhibition of ASK1 by Pyridin-2-yl-urea, the downstream signaling pathways, such as the JNK and p38 MAP kinase pathways, may be affected . These pathways are involved in various cellular processes, including inflammation, cell differentiation, cell growth, and apoptosis . Therefore, the inhibition of ASK1 could potentially lead to the modulation of these processes .
Pharmacokinetics
The pyridine moiety in the structure of the compound is known to influence basic properties such as solubility, hydrophilicity, and permeability , which could potentially affect the bioavailability of the compound.
Result of Action
The molecular and cellular effects of Pyridin-2-yl-urea’s action are largely dependent on its inhibition of ASK1 and the subsequent modulation of downstream signaling pathways . For instance, the compound’s action could potentially lead to the suppression of stress-induced apoptosis, thereby promoting cell survival .
Action Environment
The action, efficacy, and stability of Pyridin-2-yl-urea could be influenced by various environmental factors. For instance, the pH of the environment could potentially affect the compound’s stability and activity. Furthermore, the presence of other molecules or compounds in the environment could potentially affect the compound’s interaction with its target, ASK1 .
準備方法
Synthetic Routes and Reaction Conditions: Pyridin-2-yl-urea can be synthesized through several methods. One common approach involves the reaction of aminopyridines with isocyanates. Another method involves the metal- and column-free one-pot ammonolysis using a wide range of aryl and alkyl amines, resulting in nearly quantitative conversions .
Industrial Production Methods: Industrial production of pyridin-2-yl-urea typically involves large-scale synthesis using optimized reaction conditions to ensure high yield and purity. The use of phosgene or its equivalents in the reaction with amines is a common industrial approach .
化学反応の分析
Types of Reactions: Pyridin-2-yl-urea undergoes various chemical reactions, including:
Oxidation: The compound can be oxidized under specific conditions to form corresponding oxidized derivatives.
Reduction: Reduction reactions can yield reduced forms of the compound.
Substitution: The pyridine ring allows for substitution reactions, where different substituents can be introduced.
Common Reagents and Conditions:
Oxidation: Common oxidizing agents include hydrogen peroxide and potassium permanganate.
Reduction: Reducing agents such as sodium borohydride are often used.
Substitution: Reagents like halogens and nucleophiles are employed in substitution reactions.
Major Products: The major products formed from these reactions depend on the specific reagents and conditions used. For example, oxidation may yield pyridine N-oxide derivatives, while substitution can introduce various functional groups onto the pyridine ring .
類似化合物との比較
- Pyridin-3-yl-urea
- Pyridin-4-yl-urea
- Phenylurea
Comparison: Pyridin-2-yl-urea is unique due to the position of the urea moiety on the pyridine ring, which influences its chemical reactivity and biological activity. Compared to pyridin-3-yl-urea and pyridin-4-yl-urea, pyridin-2-yl-urea exhibits different binding affinities and selectivities towards biological targets. Phenylurea, lacking the pyridine ring, shows distinct chemical properties and applications .
生物活性
Pyridin-2-yl-urea is a compound of significant interest in medicinal chemistry, particularly for its biological activities, including anticancer properties. This article synthesizes findings from various studies to provide a comprehensive overview of its biological activity, mechanisms of action, and potential therapeutic applications.
Overview of Pyridin-2-yl-urea
Pyridin-2-yl-urea derivatives are characterized by the presence of a pyridine ring substituted with a urea functional group. This structural motif has been linked to various biological activities, including antiproliferative effects against cancer cell lines. The compound's ability to interact with biological targets makes it a candidate for drug development.
Antiproliferative Activity
Numerous studies have investigated the antiproliferative activity of pyridin-2-yl-urea derivatives against various cancer cell lines. Notably, one study reported the synthesis and evaluation of a series of pyridin-2-yl ureas that demonstrated significant cytotoxic effects on lung cancer cell lines (A549, NCI-H460, NCI-H1975) with IC50 values indicating effective inhibition of cell growth:
Compound | Cell Line | IC50 (μM) | Selectivity |
---|---|---|---|
Cu(U3)₂Cl₂ | NCI-H1975 | 39.6 ± 4.5 | Moderate towards WI-26 VA4 |
Cu(U11)₂Cl₂ | NCI-H1975 | 33.4 ± 3.8 | Moderate towards WI-26 VA4 |
These compounds exhibited enhanced activity against drug-resistant cells, suggesting their potential as effective anticancer agents .
The mechanism underlying the antiproliferative effects of pyridin-2-yl urea derivatives has been explored through various assays:
- Cell Cycle Analysis : Studies indicated that these compounds can arrest the cell cycle at the G1 phase, thereby inhibiting cell proliferation .
- Apoptosis Induction : Evidence suggests that certain pyridin-2-yl urea-based complexes induce apoptosis in cancer cells, contributing to their cytotoxic effects .
Synthesis and Structure Activity Relationship (SAR)
The synthesis of pyridin-2-yl urea derivatives has been optimized through various methodologies, including solvent-free reactions that enhance yield and reduce environmental impact . The structure–activity relationship (SAR) studies have identified key substituents on the pyridine ring that influence biological activity:
Substituent Position | Type | Effect on Activity |
---|---|---|
2 | Nitro | Increased potency |
4 | Methyl | Moderate potency |
5 | Chlorine | Reduced activity |
These findings emphasize the importance of specific functional groups in modulating the biological efficacy of pyridin-2-yl urea derivatives .
Case Studies
Several case studies highlight the potential therapeutic applications of pyridin-2-yl urea derivatives:
- Lung Cancer Treatment : A study focused on Cu(II) complexes derived from pyridin-2-yl urea showed promising results against lung cancer cells, with specific complexes demonstrating significant selectivity towards cancerous cells over normal cells .
- VEGFR Inhibition : Some derivatives exhibited inhibitory activity against Vascular Endothelial Growth Factor Receptor 2 (VEGFR-2), a critical target in cancer therapy, with micromolar IC50 values indicating their potential as antiangiogenic agents .
特性
IUPAC Name |
pyridin-2-ylurea | |
---|---|---|
Source | PubChem | |
URL | https://pubchem.ncbi.nlm.nih.gov | |
Description | Data deposited in or computed by PubChem | |
InChI |
InChI=1S/C6H7N3O/c7-6(10)9-5-3-1-2-4-8-5/h1-4H,(H3,7,8,9,10) | |
Source | PubChem | |
URL | https://pubchem.ncbi.nlm.nih.gov | |
Description | Data deposited in or computed by PubChem | |
InChI Key |
MQDVUDAZJMZQMF-UHFFFAOYSA-N | |
Source | PubChem | |
URL | https://pubchem.ncbi.nlm.nih.gov | |
Description | Data deposited in or computed by PubChem | |
Canonical SMILES |
C1=CC=NC(=C1)NC(=O)N | |
Source | PubChem | |
URL | https://pubchem.ncbi.nlm.nih.gov | |
Description | Data deposited in or computed by PubChem | |
Molecular Formula |
C6H7N3O | |
Source | PubChem | |
URL | https://pubchem.ncbi.nlm.nih.gov | |
Description | Data deposited in or computed by PubChem | |
DSSTOX Substance ID |
DTXSID20927088 | |
Record name | N-Pyridin-2-ylcarbamimidic acid | |
Source | EPA DSSTox | |
URL | https://comptox.epa.gov/dashboard/DTXSID20927088 | |
Description | DSSTox provides a high quality public chemistry resource for supporting improved predictive toxicology. | |
Molecular Weight |
137.14 g/mol | |
Source | PubChem | |
URL | https://pubchem.ncbi.nlm.nih.gov | |
Description | Data deposited in or computed by PubChem | |
CAS No. |
13114-65-3, 13114-64-2 | |
Record name | Urea, (3-pyridyl)- | |
Source | ChemIDplus | |
URL | https://pubchem.ncbi.nlm.nih.gov/substance/?source=chemidplus&sourceid=0013114653 | |
Description | ChemIDplus is a free, web search system that provides access to the structure and nomenclature authority files used for the identification of chemical substances cited in National Library of Medicine (NLM) databases, including the TOXNET system. | |
Record name | N-Pyridin-2-ylcarbamimidic acid | |
Source | EPA DSSTox | |
URL | https://comptox.epa.gov/dashboard/DTXSID20927088 | |
Description | DSSTox provides a high quality public chemistry resource for supporting improved predictive toxicology. | |
Record name | 13114-64-2 | |
Source | European Chemicals Agency (ECHA) | |
URL | https://echa.europa.eu/information-on-chemicals | |
Description | The European Chemicals Agency (ECHA) is an agency of the European Union which is the driving force among regulatory authorities in implementing the EU's groundbreaking chemicals legislation for the benefit of human health and the environment as well as for innovation and competitiveness. | |
Explanation | Use of the information, documents and data from the ECHA website is subject to the terms and conditions of this Legal Notice, and subject to other binding limitations provided for under applicable law, the information, documents and data made available on the ECHA website may be reproduced, distributed and/or used, totally or in part, for non-commercial purposes provided that ECHA is acknowledged as the source: "Source: European Chemicals Agency, http://echa.europa.eu/". Such acknowledgement must be included in each copy of the material. ECHA permits and encourages organisations and individuals to create links to the ECHA website under the following cumulative conditions: Links can only be made to webpages that provide a link to the Legal Notice page. | |
Retrosynthesis Analysis
AI-Powered Synthesis Planning: Our tool employs the Template_relevance Pistachio, Template_relevance Bkms_metabolic, Template_relevance Pistachio_ringbreaker, Template_relevance Reaxys, Template_relevance Reaxys_biocatalysis model, leveraging a vast database of chemical reactions to predict feasible synthetic routes.
One-Step Synthesis Focus: Specifically designed for one-step synthesis, it provides concise and direct routes for your target compounds, streamlining the synthesis process.
Accurate Predictions: Utilizing the extensive PISTACHIO, BKMS_METABOLIC, PISTACHIO_RINGBREAKER, REAXYS, REAXYS_BIOCATALYSIS database, our tool offers high-accuracy predictions, reflecting the latest in chemical research and data.
Strategy Settings
Precursor scoring | Relevance Heuristic |
---|---|
Min. plausibility | 0.01 |
Model | Template_relevance |
Template Set | Pistachio/Bkms_metabolic/Pistachio_ringbreaker/Reaxys/Reaxys_biocatalysis |
Top-N result to add to graph | 6 |
Feasible Synthetic Routes
A: Pyridin-2-yl-urea derivatives often act as negative allosteric modulators (NAMs), binding to specific sites on target proteins distinct from the active site. This binding indirectly alters the protein's conformation, impacting its activity. For instance, certain pyridin-2-yl-ureas exhibit high affinity for glycogen synthase kinase 3 (GSK-3) [], a key enzyme implicated in various neurological disorders, and act as potent inhibitors []. This inhibition could potentially modulate downstream signaling pathways involved in glycogen synthesis and other cellular processes. Another example is their activity as mGlu5 NAMs [], affecting glutamatergic neurotransmission and potentially influencing behaviors related to addiction.
A: The core structure consists of a pyridine ring substituted at the 2-position with a urea group (-NH-CO-NH-). The molecular formula and weight vary greatly depending on the specific substituents added to the core. For example, 1-(3-methylsulfanyl-phenyl)-3-pyridin-2-yl-urea (L) has a molecular formula of C13H13N3OS and a molecular weight of 259.32 g/mol []. Spectroscopic data like 1H NMR, 13C NMR, and HRMS are crucial for structural confirmation and can vary based on substituents []. For instance, the urea NH protons typically appear as distinct signals in the 1H NMR spectrum and are sensitive to hydrogen bonding interactions [, ].
A: While specific data on material compatibility is limited in the provided research, the stability of pyridin-2-yl-urea derivatives under various conditions is crucial for their applications. For example, the fluorous pyridin-2-yl-urea derivative studied by Turner et al. [] demonstrated self-association in specific solvents, highlighting the impact of environmental factors on their behavior. Understanding their stability profile in different solvents, temperatures, and pH ranges is crucial for developing suitable formulations and ensuring consistent performance in various applications, including biological assays and potential therapeutic interventions.
A: Research has explored the use of pyridin-2-yl-urea derivatives as ligands in metal-catalyzed reactions. For example, a copper(I) iodide complex immobilized on SBA-15 and functionalized with 1,1-di(pyridin-2-yl)urea exhibits catalytic activity in the aerobic oxidation of primary alcohols to aldehydes []. The urea functionality likely plays a role in coordinating the copper center and influencing its reactivity. Further research is needed to fully elucidate the reaction mechanism and explore the selectivity of these catalysts towards different substrates.
A: Density functional theory (DFT) calculations have been employed to investigate the hydrogen bonding interactions of tetraazaanthracenedione with pyridin-2-yl-urea derivatives []. These calculations provide insights into the strength and geometry of these interactions, explaining observed trends in binding affinities. QSAR models, correlating molecular structure to biological activity, could be developed using computational descriptors derived from these calculations and experimental data. Such models can guide the design of novel pyridin-2-yl-urea derivatives with improved potency and selectivity for specific targets.
A: SAR studies are crucial for optimizing the biological activity of pyridin-2-yl-urea compounds. Research highlights that the position and nature of substituents on both the pyridine and urea moieties significantly impact their binding affinity and selectivity []. For example, introducing a fluorous chain can enhance solubility in certain solvents and influence self-association behavior []. Modifications to the urea nitrogen atoms can also affect hydrogen bonding interactions with target proteins, influencing their potency and selectivity.
ANone: Ensuring stability under various conditions is crucial for the development of pyridin-2-yl-urea compounds as potential therapeutics. Degradation pathways need to be identified and mitigated through appropriate formulation strategies. Strategies to improve solubility, such as the use of co-solvents, surfactants, or cyclodextrin complexes, might be necessary for poorly soluble derivatives. Prodrug approaches, where a labile group is attached to improve bioavailability, could also be explored. Further research is needed to determine the optimal formulation approaches for specific pyridin-2-yl-urea derivatives based on their physicochemical properties and intended applications.
A: Various analytical techniques are crucial for studying pyridin-2-yl-urea compounds. NMR spectroscopy (1H and 13C) is routinely used for structural characterization, identifying characteristic signals from the pyridine and urea moieties [, , ]. High-resolution mass spectrometry (HRMS) provides accurate mass measurements, confirming the molecular formula []. For studying interactions with biological targets, techniques like X-ray crystallography [, , ] can provide detailed structural information on binding modes. Additionally, methods like HPLC coupled with UV or mass spectrometry detectors are likely used for separation and quantification in complex mixtures, though specific details are not provided in the abstracts.
A: Several synthetic routes to pyridin-2-yl-urea derivatives have been reported. A palladium-catalyzed ureidation reaction allows for the regioselective preparation of these compounds from 2-chloropyridines with good yields [, ]. This method utilizes xantphos as a ligand and offers flexibility for incorporating both aryl and alkyl ureas into the desired structure. Alternative methods using 2-aminopyridinium salts as starting materials have also been explored []. Furthermore, metal-free, one-pot protocols for synthesizing unsymmetrical ureas bearing 2-pyridyl units have been developed, expanding the accessible chemical space for these compounds [].
試験管内研究製品の免責事項と情報
BenchChemで提示されるすべての記事および製品情報は、情報提供を目的としています。BenchChemで購入可能な製品は、生体外研究のために特別に設計されています。生体外研究は、ラテン語の "in glass" に由来し、生物体の外で行われる実験を指します。これらの製品は医薬品または薬として分類されておらず、FDAから任何の医療状態、病気、または疾患の予防、治療、または治癒のために承認されていません。これらの製品を人間または動物に体内に導入する形態は、法律により厳格に禁止されています。これらのガイドラインに従うことは、研究と実験において法的および倫理的な基準の遵守を確実にするために重要です。