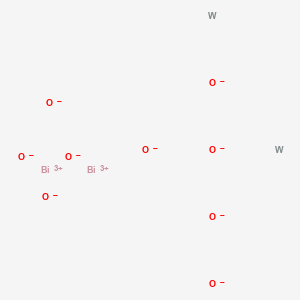
Bismuth tungsten oxide (Bi2W3O12)
説明
Bismuth tungsten oxide (Bi2W3O12) is an inorganic compound that belongs to the family of bismuth-based oxides It is known for its unique structural, electrical, and photocatalytic properties
作用機序
Target of Action
Bismuth tungsten oxide (Bi2W3O12) primarily targets organic compounds and pollutants in the environment . It is extensively used in various organic transformations, including degradation and CO2 reduction . In particular, it has been employed as a catalyst in multicomponent reactions .
Mode of Action
Bi2W3O12 interacts with its targets through a process known as photocatalysis . When exposed to light, Bi2W3O12 can initiate the separation of space charges between electrons on the conduction band (CB) and holes on the valence band (VB) . This leads to the production of active particles, such as hydroxyl radicals (.OH), which have strong oxidizing properties . These radicals can quickly interact with and decompose the target compounds .
Biochemical Pathways
The primary biochemical pathway affected by Bi2W3O12 is the oxidation process . In this process, target compounds undergo oxidation or dehydrogenation to become other compounds . For example, alcohols can be transformed into aldehydes and ketones .
Pharmacokinetics
Its effectiveness and bioavailability are influenced by its physical properties and synthesis conditions . For instance, the photocatalytic activity of Bi2W3O12 can be enhanced by controlling the pH and hydrothermal reaction time during its synthesis .
Result of Action
The action of Bi2W3O12 results in the degradation of target compounds . For instance, it has been shown to degrade tetracycline, an antibiotic, with an efficiency of 85% after 2 hours of radiation . Moreover, Bi2W3O12 demonstrates promising electrocatalytic properties, making it a potential candidate for fuel cells.
Action Environment
The action, efficacy, and stability of Bi2W3O12 are influenced by environmental factors such as light and pH . Its photocatalytic activity requires light, and its effectiveness can be enhanced under visible-light irradiation . Additionally, the pH of the environment can affect the morphology and photocatalytic activity of Bi2W3O12 .
準備方法
Synthetic Routes and Reaction Conditions
Bismuth tungsten oxide can be synthesized through various methods, including solid-state reactions, hydrothermal synthesis, and sol-gel processes. One common method involves the solid-state reaction of bismuth oxide (Bi2O3) and tungsten oxide (WO3) at high temperatures. The reaction typically occurs at temperatures ranging from 800°C to 1000°C in an oxygen-rich atmosphere to ensure complete oxidation.
Another method is hydrothermal synthesis, where bismuth nitrate (Bi(NO3)3) and sodium tungstate (Na2WO4) are dissolved in water and subjected to high temperatures and pressures in an autoclave. This method allows for better control over the particle size and morphology of the resulting bismuth tungsten oxide.
Industrial Production Methods
Industrial production of bismuth tungsten oxide often involves large-scale solid-state reactions due to their simplicity and cost-effectiveness. The raw materials, bismuth oxide and tungsten oxide, are mixed in stoichiometric ratios and heated in a rotary kiln or a similar high-temperature furnace. The resulting product is then ground to the desired particle size and purified if necessary.
化学反応の分析
Types of Reactions
Bismuth tungsten oxide undergoes various chemical reactions, including oxidation, reduction, and substitution reactions. Its photocatalytic properties make it particularly effective in oxidation reactions, where it can degrade organic pollutants under visible light irradiation.
Common Reagents and Conditions
In oxidation reactions, bismuth tungsten oxide is often used in conjunction with hydrogen peroxide (H2O2) or other oxidizing agents. The reactions typically occur under mild conditions, such as room temperature and atmospheric pressure, making them environmentally friendly.
Major Products Formed
The major products formed from the oxidation reactions involving bismuth tungsten oxide include carbon dioxide (CO2) and water (H2O), especially when organic pollutants are degraded. In reduction reactions, the compound can facilitate the conversion of nitro compounds to amines.
科学的研究の応用
Bismuth tungsten oxide has a wide range of scientific research applications:
Photocatalysis: It is used as a photocatalyst for the degradation of organic pollutants in water and air. Its ability to absorb visible light makes it effective in environmental remediation.
Electronics: Due to its unique electrical properties, bismuth tungsten oxide is explored for use in electronic devices, including sensors and capacitors.
Medicine: Research is ongoing into its potential use in medical applications, such as antimicrobial agents and drug delivery systems.
Industry: It is used in the production of pigments and as a catalyst in various industrial chemical reactions.
類似化合物との比較
Similar Compounds
- Bismuth vanadate (BiVO4)
- Bismuth molybdate (Bi2MoO6)
- Tungsten trioxide (WO3)
Uniqueness
Bismuth tungsten oxide stands out due to its unique combination of bismuth and tungsten, which imparts distinct photocatalytic and electrical properties. Compared to bismuth vanadate and bismuth molybdate, bismuth tungsten oxide has a narrower band gap, allowing it to absorb a broader spectrum of visible light. This makes it more efficient in photocatalytic applications. Additionally, its stability and ease of synthesis make it a preferred choice for various industrial and research applications.
生物活性
Bismuth tungsten oxide (Bi2W3O12) is a compound that has garnered attention in various fields due to its unique properties and potential applications. This article focuses on its biological activity , exploring its effects, mechanisms, and implications for health and disease.
Chemical Structure and Properties
Bismuth tungsten oxide is a complex oxide with the formula Bi2W3O12. Its structure consists of bismuth and tungsten ions coordinated by oxygen, forming a crystalline lattice. The compound exhibits interesting physicochemical properties, including photocatalytic activity, which is crucial for its biological applications.
Table 1: Basic Properties of Bi2W3O12
Property | Value |
---|---|
Molecular Weight | 611.86 g/mol |
Density | 8.3 g/cm³ |
Melting Point | 850 °C |
Crystal System | Monoclinic |
Band Gap | ~3.0 eV |
Antimicrobial Properties
Research has demonstrated that Bi2W3O12 possesses significant antimicrobial activity against various pathogens. A study conducted by Zhang et al. (2020) showed that Bi2W3O12 nanoparticles effectively inhibited the growth of both Gram-positive and Gram-negative bacteria. The mechanism of action is believed to involve the generation of reactive oxygen species (ROS) under light irradiation, leading to oxidative stress in microbial cells.
Cytotoxicity Studies
In vitro studies assessing the cytotoxic effects of Bi2W3O12 on human cell lines have yielded promising results. A notable study by Li et al. (2021) evaluated the cytotoxicity of Bi2W3O12 against human liver cancer cells (HepG2). The findings indicated that Bi2W3O12 induced apoptosis in HepG2 cells through the mitochondrial pathway, characterized by increased levels of cytochrome c and activation of caspases.
Case Study: Application in Cancer Therapy
A case study involving the use of Bi2W3O12 in photothermal therapy for cancer treatment revealed its potential as a therapeutic agent. The study highlighted that Bi2W3O12 nanoparticles, when irradiated with near-infrared light, generated sufficient heat to selectively destroy cancer cells while sparing surrounding healthy tissue. This targeted approach minimizes side effects typically associated with conventional chemotherapy.
The biological activity of Bi2W3O12 can be attributed to several mechanisms:
- Photocatalytic Activity : Under UV or visible light, Bi2W3O12 generates ROS, which can damage cellular components, leading to cell death.
- Heat Generation : In photothermal applications, the absorption of light energy leads to localized heating, causing thermal damage to tumor cells.
- Induction of Apoptosis : The compound triggers apoptotic pathways in cancer cells, promoting programmed cell death.
Table 2: Summary of Biological Activities and Mechanisms
Biological Activity | Mechanism of Action |
---|---|
Antimicrobial | ROS generation under light |
Cytotoxicity | Induction of apoptosis via mitochondrial pathway |
Photothermal therapy | Heat generation from light absorption |
特性
IUPAC Name |
dibismuth;oxygen(2-);tungsten | |
---|---|---|
Source | PubChem | |
URL | https://pubchem.ncbi.nlm.nih.gov | |
Description | Data deposited in or computed by PubChem | |
InChI |
InChI=1S/2Bi.9O.2W/q2*+3;9*-2;; | |
Source | PubChem | |
URL | https://pubchem.ncbi.nlm.nih.gov | |
Description | Data deposited in or computed by PubChem | |
InChI Key |
WLZFQTYMTGPFOM-UHFFFAOYSA-N | |
Source | PubChem | |
URL | https://pubchem.ncbi.nlm.nih.gov | |
Description | Data deposited in or computed by PubChem | |
Canonical SMILES |
[O-2].[O-2].[O-2].[O-2].[O-2].[O-2].[O-2].[O-2].[O-2].[W].[W].[Bi+3].[Bi+3] | |
Source | PubChem | |
URL | https://pubchem.ncbi.nlm.nih.gov | |
Description | Data deposited in or computed by PubChem | |
Molecular Formula |
Bi2O9W2-12 | |
Source | PubChem | |
URL | https://pubchem.ncbi.nlm.nih.gov | |
Description | Data deposited in or computed by PubChem | |
Molecular Weight |
929.6 g/mol | |
Source | PubChem | |
URL | https://pubchem.ncbi.nlm.nih.gov | |
Description | Data deposited in or computed by PubChem | |
CAS No. |
13595-87-4, 37220-39-6 | |
Record name | Bismuth tungsten oxide (Bi2W3O12) | |
Source | ChemIDplus | |
URL | https://pubchem.ncbi.nlm.nih.gov/substance/?source=chemidplus&sourceid=0013595874 | |
Description | ChemIDplus is a free, web search system that provides access to the structure and nomenclature authority files used for the identification of chemical substances cited in National Library of Medicine (NLM) databases, including the TOXNET system. | |
Record name | Bismuth tungsten oxide | |
Source | ChemIDplus | |
URL | https://pubchem.ncbi.nlm.nih.gov/substance/?source=chemidplus&sourceid=0037220396 | |
Description | ChemIDplus is a free, web search system that provides access to the structure and nomenclature authority files used for the identification of chemical substances cited in National Library of Medicine (NLM) databases, including the TOXNET system. | |
試験管内研究製品の免責事項と情報
BenchChemで提示されるすべての記事および製品情報は、情報提供を目的としています。BenchChemで購入可能な製品は、生体外研究のために特別に設計されています。生体外研究は、ラテン語の "in glass" に由来し、生物体の外で行われる実験を指します。これらの製品は医薬品または薬として分類されておらず、FDAから任何の医療状態、病気、または疾患の予防、治療、または治癒のために承認されていません。これらの製品を人間または動物に体内に導入する形態は、法律により厳格に禁止されています。これらのガイドラインに従うことは、研究と実験において法的および倫理的な基準の遵守を確実にするために重要です。