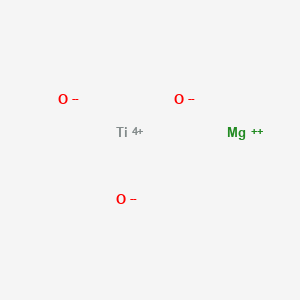
三酸化マグネシウムチタン
説明
Magnesium titanium trioxide (MgTiO3) is a ceramic material with unique properties and applications primarily in the field of electronics and ceramics. It is known for its high dielectric constant, making it suitable for microwave resonators and dielectric antenna applications.
Synthesis Analysis
MgTiO3 can be synthesized using magnesium and titanium alkoxides. The synthesis process involves the interaction between magnesium and titanium alkoxides under specific conditions to form magnesium titanate powders. The phase purity of MgTiO3 is significantly dependent on the hydrolysis conditions, and the final product can be sintered into dense ceramics at relatively lower temperatures compared to conventional methods (Yanovskaya et al., 1998).
Molecular Structure Analysis
The molecular structure of MgTiO3 includes a statistical distribution of heteroatoms within the metal alkoxides framework. This unique structure contributes to its chemical and physical properties, which are crucial for its applications in various technological fields.
Chemical Reactions and Properties
MgTiO3 undergoes various chemical reactions based on its composition and environmental conditions. The synthesis and stability of MgTiO3 are influenced by reactions between boron and titanium compounds with magnesium, leading to the formation of magnesium and titanium borides through a series of reactions (Matin et al., 2001).
科学的研究の応用
生体医用インプラント
三酸化マグネシウムチタン複合材料は、近年、部分的に分解可能な生体材料として注目を集めています . チタン-マグネシウム複合材料は、マグネシウムの生物活性とチタンの良好な機械的特性を兼ね備えています . これらの複合材料は生体医用インプラントに使用され、その製造方法は浸透鋳造、粉末冶金、熱間回転圧延などがあります .
電子機器とセラミックス
三酸化マグネシウムチタンは、主に電子機器とセラミックスの分野でユニークな特性と用途を持つセラミック材料です. それは高い誘電率で知られており、マイクロ波共振器や誘電体アンテナの用途に適しています.
エネルギー貯蔵
エネルギー貯蔵の分野では、MgTiO3はマグネシウム系水素化物における触媒として使用されてきました. これらの水素化物は、固体水素貯蔵および熱エネルギー貯蔵のための有望な候補と見なされています.
ナノテクノロジー
三酸化マグネシウムチタンは、ナノマテリアルの分野で潜在的な用途があります. MgTiO3のユニークな分子構造は、さまざまな技術分野における用途に不可欠な化学的および物理的特性に寄与しています.
生体医科学
生体医科学の分野では、MgTiO3は潜在的な用途があります. MgTiO3の合成と安定性は、ホウ素とチタン化合物のマグネシウムとの反応によって影響を受けます.
化学工学
三酸化マグネシウムチタンは、さまざまな化学反応において触媒として使用されてきました. 合成プロセスには、特定の条件下でマグネシウムとチタンアルコキシドの相互作用が含まれ、チタン酸マグネシウム粉末が形成されます.
材料工学
マグネシウムは、MgTiO3などの化合物とともに、自動車、航空宇宙、家電製品などの工学用途に使用されています. 最終製品は、従来の方法と比較して比較的低い温度で緻密なセラミックスに焼結できます.
作用機序
Target of Action
Magnesium titanium trioxide (MgTiO3) is primarily used in the field of electronics and ceramics. It is known for its high dielectric constant, making it suitable for microwave resonators and dielectric antenna applications. It has also been used as a catalyst in magnesium-based hydrides, which are considered promising candidates for solid-state hydrogen storage and thermal energy storage .
Action Environment
The action of MgTiO3 can be influenced by environmental factors. . This suggests that the efficacy and stability of MgTiO3 could also be influenced by environmental conditions, although more research is needed to confirm this.
Safety and Hazards
将来の方向性
The development of MgTiO3 family composites has become one of the main research directions in the “Hydrogen Economy” theme because of their high hydrogen storage capacity, high energy density, low cost, and good reversibility . Another promising direction is the deposition of magnesium on surface-modified titanium for potential tissue engineering applications .
特性
IUPAC Name |
magnesium;oxygen(2-);titanium(4+) | |
---|---|---|
Source | PubChem | |
URL | https://pubchem.ncbi.nlm.nih.gov | |
Description | Data deposited in or computed by PubChem | |
InChI |
InChI=1S/Mg.3O.Ti/q+2;3*-2;+4 | |
Source | PubChem | |
URL | https://pubchem.ncbi.nlm.nih.gov | |
Description | Data deposited in or computed by PubChem | |
InChI Key |
XSETZKVZGUWPFM-UHFFFAOYSA-N | |
Source | PubChem | |
URL | https://pubchem.ncbi.nlm.nih.gov | |
Description | Data deposited in or computed by PubChem | |
Canonical SMILES |
[O-2].[O-2].[O-2].[Mg+2].[Ti+4] | |
Source | PubChem | |
URL | https://pubchem.ncbi.nlm.nih.gov | |
Description | Data deposited in or computed by PubChem | |
Molecular Formula |
MgO3Ti | |
Source | PubChem | |
URL | https://pubchem.ncbi.nlm.nih.gov | |
Description | Data deposited in or computed by PubChem | |
DSSTOX Substance ID |
DTXSID801340590 | |
Record name | Geikielite (Mg(TiO3)) | |
Source | EPA DSSTox | |
URL | https://comptox.epa.gov/dashboard/DTXSID801340590 | |
Description | DSSTox provides a high quality public chemistry resource for supporting improved predictive toxicology. | |
Molecular Weight |
120.17 g/mol | |
Source | PubChem | |
URL | https://pubchem.ncbi.nlm.nih.gov | |
Description | Data deposited in or computed by PubChem | |
CAS RN |
12032-30-3, 12032-35-8, 1312-99-8 | |
Record name | Magnesium titanium oxide (MgTiO3) | |
Source | ChemIDplus | |
URL | https://pubchem.ncbi.nlm.nih.gov/substance/?source=chemidplus&sourceid=0012032303 | |
Description | ChemIDplus is a free, web search system that provides access to the structure and nomenclature authority files used for the identification of chemical substances cited in National Library of Medicine (NLM) databases, including the TOXNET system. | |
Record name | Magnesium titanium oxide (MgTi2O5) | |
Source | ChemIDplus | |
URL | https://pubchem.ncbi.nlm.nih.gov/substance/?source=chemidplus&sourceid=0012032358 | |
Description | ChemIDplus is a free, web search system that provides access to the structure and nomenclature authority files used for the identification of chemical substances cited in National Library of Medicine (NLM) databases, including the TOXNET system. | |
Record name | Geikielite (Mg(TiO3)) | |
Source | EPA Chemicals under the TSCA | |
URL | https://www.epa.gov/chemicals-under-tsca | |
Description | EPA Chemicals under the Toxic Substances Control Act (TSCA) collection contains information on chemicals and their regulations under TSCA, including non-confidential content from the TSCA Chemical Substance Inventory and Chemical Data Reporting. | |
Record name | Geikielite (Mg(TiO3)) | |
Source | EPA DSSTox | |
URL | https://comptox.epa.gov/dashboard/DTXSID801340590 | |
Description | DSSTox provides a high quality public chemistry resource for supporting improved predictive toxicology. | |
Record name | Magnesium dititanate | |
Source | European Chemicals Agency (ECHA) | |
URL | https://echa.europa.eu/substance-information/-/substanceinfo/100.031.596 | |
Description | The European Chemicals Agency (ECHA) is an agency of the European Union which is the driving force among regulatory authorities in implementing the EU's groundbreaking chemicals legislation for the benefit of human health and the environment as well as for innovation and competitiveness. | |
Explanation | Use of the information, documents and data from the ECHA website is subject to the terms and conditions of this Legal Notice, and subject to other binding limitations provided for under applicable law, the information, documents and data made available on the ECHA website may be reproduced, distributed and/or used, totally or in part, for non-commercial purposes provided that ECHA is acknowledged as the source: "Source: European Chemicals Agency, http://echa.europa.eu/". Such acknowledgement must be included in each copy of the material. ECHA permits and encourages organisations and individuals to create links to the ECHA website under the following cumulative conditions: Links can only be made to webpages that provide a link to the Legal Notice page. | |
試験管内研究製品の免責事項と情報
BenchChemで提示されるすべての記事および製品情報は、情報提供を目的としています。BenchChemで購入可能な製品は、生体外研究のために特別に設計されています。生体外研究は、ラテン語の "in glass" に由来し、生物体の外で行われる実験を指します。これらの製品は医薬品または薬として分類されておらず、FDAから任何の医療状態、病気、または疾患の予防、治療、または治癒のために承認されていません。これらの製品を人間または動物に体内に導入する形態は、法律により厳格に禁止されています。これらのガイドラインに従うことは、研究と実験において法的および倫理的な基準の遵守を確実にするために重要です。