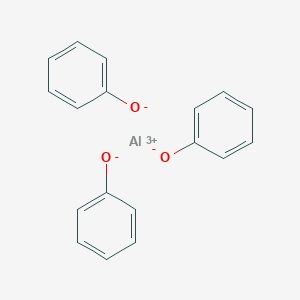
Aluminum phenoxide
概要
説明
Aluminum phenoxide, also known as aluminium phenolate, is a metalloorganic compound with the formula [Al(OC₆H₅)₃]ₙ. It is a white solid that is commonly used as a catalyst in various chemical reactions. The compound is known for its ability to form stable complexes and is often utilized in the synthesis of organic compounds .
準備方法
Synthetic Routes and Reaction Conditions: Aluminum phenoxide can be synthesized by reacting elemental aluminium with phenol. The reaction typically occurs under a nitrogen atmosphere to prevent oxidation. The process involves heating phenol to around 165°C and then gradually adding aluminium turnings with vigorous stirring : [ \text{Al} + 3 \text{HOC}_6\text{H}_5 \rightarrow \text{Al(OC}_6\text{H}_5\text{)}_3 + 1.5 \text{H}_2 ]
Industrial Production Methods: In industrial settings, aluminium triphenolate is produced using similar methods but on a larger scale. The reaction is carried out in high-pressure autoclaves to ensure complete conversion of the reactants. The product is then purified through crystallization and filtration processes .
化学反応の分析
Types of Reactions: Aluminum phenoxide undergoes various types of chemical reactions, including:
Oxidation: It can be oxidized to form aluminium oxide and phenol.
Reduction: It can be reduced back to elemental aluminium and phenol under specific conditions.
Substitution: It can participate in substitution reactions where the phenolate ligands are replaced by other ligands.
Common Reagents and Conditions:
Oxidation: Oxygen or other oxidizing agents.
Reduction: Hydrogen or other reducing agents.
Substitution: Various ligands such as halides or alkoxides.
Major Products Formed:
Oxidation: Aluminium oxide and phenol.
Reduction: Elemental aluminium and phenol.
Substitution: Aluminium complexes with different ligands.
科学的研究の応用
Catalytic Applications
Aluminum phenoxide is widely recognized for its role as a catalyst in organic reactions. Its ability to facilitate various chemical transformations makes it invaluable in both industrial and laboratory settings.
1.1. Phenolic Alkylation
One of the primary applications of this compound is in the alkylation of phenols. The compound acts as a catalyst to enhance the reaction efficiency between phenols and olefins, leading to the formation of alkylated phenolic compounds. A notable patent describes a method for removing this compound from a phenolic alkylation mixture, which demonstrates its utility in industrial processes .
Case Study:
- Reaction: Alkylation of phenol with isobutylene using this compound.
- Outcome: The process yielded high selectivity for ortho-alkylated products, showcasing the effectiveness of this compound as a catalyst.
Polymerization Initiator
This compound has been studied as an initiator for polymerization processes, particularly in the ring-opening polymerization of cyclic monomers such as epoxides and lactones.
2.1. Reactivity in Polymerization
Research indicates that this compound can initiate the polymerization of epoxides and β-lactones effectively. The reactivity order was found to be epoxide > β-lactone > ε-lactone, highlighting its potential for synthesizing various polymer materials .
Data Table: Polymerization Reactivity
Monomer Type | Reactivity Order |
---|---|
Epoxide | Highest |
β-Lactone | Moderate |
ε-Lactone | Lowest |
Case Study:
- Study: Yasuda et al. explored the initiation of polymerization using aluminum porphyrin derivatives containing this compound.
- Findings: The study revealed that this compound-based catalysts could lead to high molecular weight polymers with controlled architectures .
Synthesis Reagent
This compound serves as a reagent in various organic synthesis reactions, particularly in the formation of complex organic molecules.
3.1. Hydroalkoxylation Reactions
The compound has been utilized in hydroalkoxylation reactions, where it facilitates the addition of alcohols to alkenes under elevated temperatures. This application is particularly useful for synthesizing coumarans and other oxygen heterocycles .
Case Study:
- Reaction: Hydroalkoxylation of diene compounds using this compound.
- Outcome: The reaction produced coumaran derivatives efficiently, demonstrating its utility as a synthetic reagent.
Structural Characterization and Behavior
Understanding the structural characteristics of this compound is crucial for optimizing its applications. Studies utilizing single-crystal X-ray diffraction and NMR spectroscopy have elucidated its molecular geometry and behavior in different solvents .
Key Findings:
- This compound exists predominantly as dimeric structures in solid-state.
- In solution, it exhibits four- and five-coordinate species depending on solvent interactions.
作用機序
The mechanism of action of aluminium triphenolate involves the formation of stable complexes with various substrates. The aluminium center acts as a Lewis acid, facilitating the activation of the phenolate ligands. This activation allows for various chemical transformations, such as alkylation and substitution reactions .
類似化合物との比較
Aluminium isopropoxide: Another aluminium-based compound used as a catalyst in organic synthesis.
Aluminium ethoxide: Similar in structure and reactivity but with ethoxide ligands instead of phenolate.
Aluminium tert-butoxide: Used in similar applications but with tert-butoxide ligands.
Uniqueness: Aluminum phenoxide is unique due to its ability to form stable complexes with phenolate ligands, making it particularly effective in catalyzing alkylation reactions. Its stability and reactivity make it a valuable compound in both research and industrial applications .
生物活性
Aluminum phenoxide, a compound formed from aluminum and phenolic compounds, has garnered attention for its diverse biological activities and potential applications in medicinal chemistry, catalysis, and materials science. This article explores the biological activity of this compound, including its mechanisms of action, pharmacokinetics, and implications for health based on a review of recent literature.
Chemical Structure and Properties
This compound can be represented as , where aluminum is coordinated with three phenoxide groups. This compound exhibits unique properties due to the presence of the aluminum center, which can act as a Lewis acid, facilitating various biochemical interactions.
Immune Modulation
this compound is recognized for its role as an adjuvant in vaccines. It enhances the immune response by forming a depot at the injection site, leading to a prolonged release of antigens. This mechanism is crucial for improving vaccine efficacy by stimulating both humoral and cellular immunity.
Biochemical Pathways
Research indicates that aluminum compounds influence several biochemical pathways related to aluminum metabolism in humans. They affect absorption, transport, tissue distribution, and excretion of aluminum. Notably, this compound may alter the metabolism of other metals and interfere with hematopoiesis under certain conditions .
Pharmacokinetics
This compound is absorbed through the gastrointestinal tract and can accumulate in various tissues, including bones and the brain. Its pharmacokinetic profile suggests that it may pose risks at elevated exposure levels, particularly concerning neurotoxicity and potential links to neurodegenerative diseases like Alzheimer's .
Case Studies
-
Neurotoxicity in Animal Models
Studies have demonstrated that exposure to aluminum compounds can lead to neurotoxic effects, including increased amyloid plaque formation in transgenic mouse models. These findings raise concerns about the long-term implications of aluminum exposure on cognitive health . -
Hematological Effects
Research has shown that aluminum can impact red blood cell production, particularly in iron-deficient conditions. The competition between aluminum and iron for transferrin binding can exacerbate anemia .
Applications in Research and Industry
This compound has several applications across different fields:
- Catalysis : It serves as a catalyst in organic reactions such as the alkylation of phenols, facilitating the production of various derivatives .
- Drug Delivery Systems : Ongoing research is exploring its potential as a drug delivery agent due to its stable complex formation with biomolecules .
- Material Science : Its unique properties make it suitable for applications in polymer synthesis and as an additive in lubricants .
Data Table: Summary of Biological Activities
特性
IUPAC Name |
aluminum;triphenoxide | |
---|---|---|
Source | PubChem | |
URL | https://pubchem.ncbi.nlm.nih.gov | |
Description | Data deposited in or computed by PubChem | |
InChI |
InChI=1S/3C6H6O.Al/c3*7-6-4-2-1-3-5-6;/h3*1-5,7H;/q;;;+3/p-3 | |
Source | PubChem | |
URL | https://pubchem.ncbi.nlm.nih.gov | |
Description | Data deposited in or computed by PubChem | |
InChI Key |
OPSWAWSNPREEFQ-UHFFFAOYSA-K | |
Source | PubChem | |
URL | https://pubchem.ncbi.nlm.nih.gov | |
Description | Data deposited in or computed by PubChem | |
Canonical SMILES |
C1=CC=C(C=C1)[O-].C1=CC=C(C=C1)[O-].C1=CC=C(C=C1)[O-].[Al+3] | |
Source | PubChem | |
URL | https://pubchem.ncbi.nlm.nih.gov | |
Description | Data deposited in or computed by PubChem | |
Molecular Formula |
C18H15AlO3 | |
Source | PubChem | |
URL | https://pubchem.ncbi.nlm.nih.gov | |
Description | Data deposited in or computed by PubChem | |
DSSTOX Substance ID |
DTXSID401015343 | |
Record name | Phenol, aluminum salt (3:1) | |
Source | EPA DSSTox | |
URL | https://comptox.epa.gov/dashboard/DTXSID401015343 | |
Description | DSSTox provides a high quality public chemistry resource for supporting improved predictive toxicology. | |
Molecular Weight |
306.3 g/mol | |
Source | PubChem | |
URL | https://pubchem.ncbi.nlm.nih.gov | |
Description | Data deposited in or computed by PubChem | |
CAS No. |
15086-27-8 | |
Record name | Phenol, aluminum salt (3:1) | |
Source | ChemIDplus | |
URL | https://pubchem.ncbi.nlm.nih.gov/substance/?source=chemidplus&sourceid=0015086278 | |
Description | ChemIDplus is a free, web search system that provides access to the structure and nomenclature authority files used for the identification of chemical substances cited in National Library of Medicine (NLM) databases, including the TOXNET system. | |
Record name | Phenol, aluminum salt (3:1) | |
Source | EPA Chemicals under the TSCA | |
URL | https://www.epa.gov/chemicals-under-tsca | |
Description | EPA Chemicals under the Toxic Substances Control Act (TSCA) collection contains information on chemicals and their regulations under TSCA, including non-confidential content from the TSCA Chemical Substance Inventory and Chemical Data Reporting. | |
Record name | Phenol, aluminum salt (3:1) | |
Source | EPA DSSTox | |
URL | https://comptox.epa.gov/dashboard/DTXSID401015343 | |
Description | DSSTox provides a high quality public chemistry resource for supporting improved predictive toxicology. | |
Record name | Aluminium triphenolate | |
Source | European Chemicals Agency (ECHA) | |
URL | https://echa.europa.eu/substance-information/-/substanceinfo/100.035.565 | |
Description | The European Chemicals Agency (ECHA) is an agency of the European Union which is the driving force among regulatory authorities in implementing the EU's groundbreaking chemicals legislation for the benefit of human health and the environment as well as for innovation and competitiveness. | |
Explanation | Use of the information, documents and data from the ECHA website is subject to the terms and conditions of this Legal Notice, and subject to other binding limitations provided for under applicable law, the information, documents and data made available on the ECHA website may be reproduced, distributed and/or used, totally or in part, for non-commercial purposes provided that ECHA is acknowledged as the source: "Source: European Chemicals Agency, http://echa.europa.eu/". Such acknowledgement must be included in each copy of the material. ECHA permits and encourages organisations and individuals to create links to the ECHA website under the following cumulative conditions: Links can only be made to webpages that provide a link to the Legal Notice page. | |
Synthesis routes and methods I
Procedure details
Synthesis routes and methods II
Procedure details
Synthesis routes and methods III
Procedure details
Synthesis routes and methods IV
Procedure details
Retrosynthesis Analysis
AI-Powered Synthesis Planning: Our tool employs the Template_relevance Pistachio, Template_relevance Bkms_metabolic, Template_relevance Pistachio_ringbreaker, Template_relevance Reaxys, Template_relevance Reaxys_biocatalysis model, leveraging a vast database of chemical reactions to predict feasible synthetic routes.
One-Step Synthesis Focus: Specifically designed for one-step synthesis, it provides concise and direct routes for your target compounds, streamlining the synthesis process.
Accurate Predictions: Utilizing the extensive PISTACHIO, BKMS_METABOLIC, PISTACHIO_RINGBREAKER, REAXYS, REAXYS_BIOCATALYSIS database, our tool offers high-accuracy predictions, reflecting the latest in chemical research and data.
Strategy Settings
Precursor scoring | Relevance Heuristic |
---|---|
Min. plausibility | 0.01 |
Model | Template_relevance |
Template Set | Pistachio/Bkms_metabolic/Pistachio_ringbreaker/Reaxys/Reaxys_biocatalysis |
Top-N result to add to graph | 6 |
Feasible Synthetic Routes
Q1: How do aluminium triphenolate catalysts contribute to the synthesis of heterocyclic compounds?
A: Aluminium triphenolates act as potent Lewis acid catalysts in facilitating the coupling reactions between epoxides and various heterocumulenes (like CO2 and SO2) []. This catalytic activity stems from the aluminum center's ability to activate epoxides, making them susceptible to nucleophilic attack by the heterocumulene. This process ultimately leads to the formation of a diverse range of valuable heterocyclic compounds, including cyclic carbonates, sulfites, and oxazolidinones [].
Q2: What makes aluminium triphenolates advantageous over other catalysts in these reactions?
A2: Aluminium triphenolates offer a unique blend of advantages as catalysts in these reactions:
- Tunability: The ligand structure, specifically the substituents on the phenolate rings, can be readily modified. This allows for fine-tuning of the catalyst's activity, selectivity, and stability [].
- Accessibility: These catalysts are easily synthesized, making them a practical choice for research and potential industrial applications [].
- Environmental Considerations: The utilization of aluminium triphenolates enables the incorporation of waste gases like CO2 and SO2 as reactants. This aligns with the growing emphasis on green chemistry principles by utilizing these environmentally harmful gases as valuable synthetic building blocks [].
Q3: Can you provide an example of how the stereoselectivity of aluminium triphenolate catalyzed reactions is controlled?
A: The research highlights the synthesis of carbamates from cyclic epoxides, CO2, and amines []. The researchers successfully controlled the diastereoselectivity (cis vs. trans product formation) by strategically manipulating the reaction conditions to favor either a cyclic carbonate or an oligocarbonate intermediate. These intermediates, due to their inherent configurational differences, ultimately dictate the stereochemical outcome of the final carbamate product []. This exemplifies the potential for fine-tuning reaction conditions to achieve desired stereochemical outcomes.
試験管内研究製品の免責事項と情報
BenchChemで提示されるすべての記事および製品情報は、情報提供を目的としています。BenchChemで購入可能な製品は、生体外研究のために特別に設計されています。生体外研究は、ラテン語の "in glass" に由来し、生物体の外で行われる実験を指します。これらの製品は医薬品または薬として分類されておらず、FDAから任何の医療状態、病気、または疾患の予防、治療、または治癒のために承認されていません。これらの製品を人間または動物に体内に導入する形態は、法律により厳格に禁止されています。これらのガイドラインに従うことは、研究と実験において法的および倫理的な基準の遵守を確実にするために重要です。