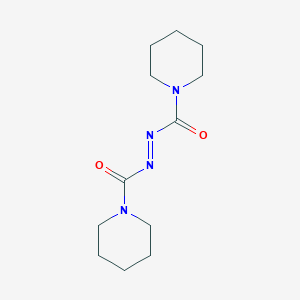
1,1'-(Azodicarbonyl)dipiperidine
概要
説明
1,1'-(Azodicarbonyl)dipiperidine (ADDP) is a stable, crystalline azo compound widely employed in organic synthesis, particularly in Mitsunobu reactions, where it serves as a redox-active reagent alongside phosphines. Its molecular formula is C₁₂H₂₀N₄O₂, with a molecular weight of 252.32 g/mol . It is valued for its ability to generate byproducts with distinct polarity, simplifying purification via column chromatography compared to traditional Mitsunobu reagents like diethyl azodicarboxylate (DEAD) . ADDP also demonstrates versatility in Diels-Alder reactions , oxidation of alcohols , and stereospecific synthesis of quaternary amino acids .
準備方法
合成経路と反応条件: 1,1’-(アゾジカルボニル)ジピペリジンは、アゾジカルボン酸とピペリジンを制御された条件下で反応させることで合成できます . この反応は、通常、エタノールやジメチルホルムアミド(DMF)などの溶媒を使用し、酸化を防ぐために不活性ガスでパージする必要があります . その後、化合物は再結晶によって精製され、高純度の生成物が得られます。
工業生産方法: 工業的には、1,1’-(アゾジカルボニル)ジピペリジンの製造は、同様の合成経路に従いますが、より大規模に行われます。 このプロセスには、自動反応器と連続フローシステムの使用が含まれ、品質と収量の均一性が確保されます . 最終製品は、業界基準を満たすために厳格な品質管理検査を受けます。
化学反応の分析
反応の種類: 1,1’-(アゾジカルボニル)ジピペリジンは、以下を含むさまざまな化学反応を起こします。
酸化: この化合物は酸化されて対応する酸化物を生成します。
還元: 還元反応によって、アゾ基はヒドラジン誘導体に変換されます。
一般的な試薬と条件:
酸化: 一般的な酸化剤には、過酸化水素と過マンガン酸カリウムがあります。
還元: 水素化ホウ素ナトリウムまたは水素化リチウムアルミニウムなどの還元剤が使用されます。
主な生成物:
酸化: 酸化物および関連化合物の生成。
還元: ヒドラジン誘導体の生成。
科学的研究の応用
Mitsunobu Reactions
ADDP serves as a key reagent in the Mitsunobu reaction, a well-established method for the inversion of alcohols and the formation of various carbon-carbon and carbon-heteroatom bonds. The efficiency of ADDP in this context has been documented in several studies:
- Modified Protocols : A modified Mitsunobu protocol utilizing ADDP and polymer-supported triphenylphosphine has shown significant improvements in reaction yields and times, facilitating the synthesis of PPAR agonists .
- Yield Data : In one study, the yields from various alcohols using ADDP ranged from 71% to 100%, demonstrating its effectiveness across different substrates (see Table 1) .
Entry | Alcohol | Product | Yield (%) |
---|---|---|---|
1 | 9 | - | 81 |
2 | 10 | - | 78 |
3 | 11 | - | 80 |
... | ... | ... | ... |
20 | 76 | - | 76 |
Synthesis of Complex Molecules
ADDP is instrumental in synthesizing complex organic molecules such as:
- Optically Active Amino Acids : Through Mitsunobu reactions, ADDP facilitates the formation of α,α-disubstituted amino acids .
- Aza-β-Lactams : The compound participates in [2+2] cycloaddition reactions to yield aza-β-lactams, which are significant in medicinal chemistry .
Medicinal Chemistry Applications
ADDP's utility extends into medicinal chemistry where it aids in the development of pharmaceutical compounds:
- Histamine H3 Receptor Antagonists : The compound has been used to synthesize histamine H3 receptor antagonists, which are potential therapeutic agents for various neurological disorders .
- Glycosyl Disulfides : It plays a role in synthesizing glycosyl disulfides that are crucial for developing neoglycopeptides .
Case Studies and Research Findings
Several studies have highlighted the applications of ADDP:
- Radiosensitization Studies : Research on the radiosensitization effects of related compounds indicates that diazenedicarboxylic acid derivatives can enhance the efficacy of radiation therapy by modifying cellular responses to radiation .
- Copper-Catalyzed Reactions : ADDP has been employed in copper-catalyzed reactions involving arylboronic acids, showcasing its versatility as a reagent under mild conditions .
作用機序
1,1’-(アゾジカルボニル)ジピペリジンの作用機序は、ミッツノブ反応における試薬としての役割に関係しています。 この化合物は、アルコールのさまざまな求核剤による求核置換を促進し、エステル、エーテル、およびその他の誘導体の生成につながります . この反応は、トリフェニルホスフィンとの中間体錯体の形成を介して進行し、その後、アルコールと求核剤による求核攻撃を受けます .
類似化合物:
アゾジカルボン酸ジエチル: ミッツノブ反応で一般的に使用されるもう1つの試薬。
アゾジカルボン酸ジエチルエステル: 1,1’-(アゾジカルボニル)ジピペリジンと構造と反応性が似ています.
独自性: 1,1’-(アゾジカルボニル)ジピペリジンは、有機合成において独自の反応性と選択性を提供する特定の構造的特徴を持つため、独自です . 高効率でミッツノブ反応を促進できるため、学術研究と産業研究の両方で貴重な試薬となっています .
類似化合物との比較
Key Structural and Functional Differences
ADDP belongs to the azo-dicarboxylate family , sharing functional similarities with DEAD and diisopropyl azodicarboxylate (DIAD). However, its piperidine substituents confer distinct advantages in substrate compatibility and reaction outcomes. Below is a comparative analysis:
Reaction Performance
Mitsunobu Reactions
- ADDP vs. DEAD/DIAD: ADDP pairs effectively with trimethylphosphine (PMe₃) to enable Mitsunobu reactions where DEAD/DIAD fail, particularly with tertiary alcohols and sterically hindered substrates. For example, ADDP-PMe₃ achieved >99% enantiospecificity in synthesizing quaternary amino acids, whereas DIAD-TPP led to elimination byproducts . DEAD is also sensitive to acidic substrates, whereas ADDP tolerates lower acidity .
- Byproduct Handling : ADDP’s reduction byproduct (1,1'-(hydrazodicarbonyl)dipiperidine ) is less polar than DEAD/DIAD byproducts, allowing straightforward column chromatography .
Oxidation and Diels-Alder Reactions
- ADDP oxidizes primary/secondary alcohols via bromide intermediates, even in the presence of sensitive functional groups (e.g., alkenes, amines) .
- In Diels-Alder reactions, ADDP acts as a dienophile, yielding bicyclic pyridazino[4,3-c]azepine derivatives in 55–77% yields .
Case Studies
Stereospecific Amino Acid Synthesis: ADDP-PMe₃ enabled the Mitsunobu reaction of chiral tertiary α-hydroxy esters with HN₃, producing α-azido esters with complete inversion of configuration. Subsequent reduction yielded α,α-disubstituted amino acids in >99% enantiomeric excess .
Cubane Alcohol Oxidation: ADDP caused decomposition of cubane alcohols under standard Mitsunobu conditions, highlighting its sensitivity to strained systems .
Neuroprotective Agent Synthesis : ADDP facilitated the preparation of PROTAC molecules targeting Alzheimer’s disease, demonstrating its utility in medicinal chemistry .
生物活性
1,1'-(Azodicarbonyl)dipiperidine, commonly referred to as ADDP, is a compound that has garnered attention for its diverse applications in organic synthesis and potential biological activities. This article explores the biological activity of ADDP, focusing on its mechanisms of action, therapeutic applications, and relevant research findings.
ADDP is characterized by its azo and carbonyl functional groups, which contribute to its reactivity in various chemical reactions. It is primarily used as a reagent in the Mitsunobu reaction, facilitating the formation of carbon-nitrogen bonds . The synthesis of ADDP typically involves the reaction of piperidine with azodicarboxylic acid derivatives under controlled conditions to optimize yield and purity.
The biological activity of ADDP is closely tied to its role as a reagent in organic synthesis. Notably, it has been employed in the synthesis of G protein-coupled receptor (GPCR) agonists, which are crucial in various physiological processes. Research indicates that compounds synthesized using ADDP can activate GPCRs associated with metabolic regulation, particularly those involved in glucose metabolism and insulin secretion .
Therapeutic Applications
ADDP's potential therapeutic applications are particularly relevant in the context of diabetes management. Studies have highlighted its role in synthesizing glucokinase (GK) activators, which enhance insulin secretion and improve glucose tolerance. GK plays a pivotal role in glucose homeostasis, and compounds derived from ADDP have shown promise in treating conditions such as type 2 diabetes and obesity .
Table 1: Summary of Biological Activities Related to ADDP
Activity | Description |
---|---|
GPCR Activation | Enhances insulin secretion through GPCR pathways |
Glucokinase Activation | Improves glucose tolerance and regulates blood sugar levels |
Antidiabetic Potential | Compounds synthesized with ADDP show efficacy in managing diabetes |
Case Studies
- Glucokinase Activators : Research has demonstrated that certain compounds synthesized using ADDP exhibit superior glucokinase activating properties. These compounds were tested in various animal models, showing significant improvements in blood glucose levels and insulin sensitivity .
- Mitsunobu Reaction Applications : ADDP has been successfully utilized in the Mitsunobu reaction to synthesize aryl-substituted hydrazides, which have been studied for their anti-diabetic effects. The efficiency of this reaction highlights ADDP's versatility as a reagent in producing biologically active compounds .
Comparative Analysis
ADDP's unique structure allows it to participate in various chemical reactions that yield biologically active products. Compared to similar compounds like diisopropyl azodicarboxylate, ADDP offers distinct advantages due to its specific functional groups that enhance its reactivity and biological potential .
Table 2: Comparison with Similar Compounds
Compound | Applications | Unique Features |
---|---|---|
This compound | GPCR agonists, glucokinase activators | Unique azo-carbonyl structure |
Diisopropyl azodicarboxylate | Organic synthesis | Less versatile than ADDP |
Azodicarboxylic acid bis(dimethylamide) | Various chemical reactions | Different functional groups |
Q & A
Basic Questions
Q. What are the primary applications of ADDP in organic synthesis?
ADDP is a reagent widely used in Mitsunobu reactions to facilitate SN2-type inversions of alcohols, enabling the formation of ethers, esters, or carbon-carbon bonds . Unlike diethyl azodicarboxylate (DEAD), ADDP is effective for substrates with lower acidity (pKa > 11) , such as tertiary alcohols or sterically hindered secondary alcohols, due to its stronger electron-donating piperidine groups . It is also employed in synthesizing agonists for GPR120 (antidiabetic targets) and PPARα/γ/δ (metabolic regulators) .
Q. What safety precautions are critical when handling ADDP?
ADDP poses hazards including skin/eye irritation (H315/H319) and respiratory toxicity (H335). Key precautions include:
- Use PPE : gloves, goggles, and lab coats .
- Work in well-ventilated areas or fume hoods to avoid inhalation .
- Store in airtight containers at -20°C (powder) or -80°C (solutions) to prevent decomposition .
- In case of exposure, rinse skin/eyes with water for 15 minutes and seek medical attention .
Advanced Research Questions
Q. How can ADDP-based Mitsunobu reactions be optimized to minimize by-product formation?
By-product formation (e.g., oxidized phosphine intermediates) can be reduced by:
- Solvent selection : Use anhydrous THF or acetonitrile to enhance reagent stability .
- Stoichiometry : Maintain a 1:1:1 ratio of ADDP, phosphine (e.g., tributylphosphine), and substrate .
- Phosphine choice : Polymer-supported triphenylphosphine (PS-PPh₃) simplifies purification and reduces phosphine oxide by-products .
- Temperature control : Reactions at 0–25°C improve selectivity for acid-sensitive substrates .
Q. How do contradictions in literature regarding ADDP’s substrate specificity arise, and how can they be resolved?
Discrepancies often stem from differences in substrate acidity and reaction conditions . For example:
- ADDP outperforms DEAD in reactions with low-acidity alcohols (pKa > 11) but may fail with highly acidic substrates (e.g., phenols) .
- Conflicting reports on reaction efficiency can be addressed by systematic pKa analysis of substrates and kinetic monitoring (e.g., via in situ NMR) to identify optimal conditions .
Q. What analytical methods are most reliable for validating ADDP’s role in synthesizing complex molecules like PPAR agonists?
Key methods include:
- NMR spectroscopy : Track the disappearance of alcohol protons and formation of new C-O/C-N bonds .
- HPLC-MS : Confirm product purity and detect trace by-products (e.g., hydrazine derivatives) .
- X-ray crystallography : Resolve stereochemical outcomes in chiral molecules synthesized via Mitsunobu .
Q. What challenges arise when using ADDP in hypoxia-targeted drug conjugates (e.g., AzCDF), and how are they mitigated?
Challenges include:
- Stability : ADDP’s sensitivity to moisture requires strict anhydrous conditions during coupling reactions .
- By-product interference : Use flash chromatography or SCX ion-exchange resins to remove hydrazine derivatives .
- Functional group compatibility : Avoid substrates with reducible groups (e.g., nitro, azides) that react with ADDP’s azo moiety .
特性
CAS番号 |
10465-81-3 |
---|---|
分子式 |
C12H20N4O2 |
分子量 |
252.31 g/mol |
IUPAC名 |
N-(piperidine-1-carbonylimino)piperidine-1-carboxamide |
InChI |
InChI=1S/C12H20N4O2/c17-11(15-7-3-1-4-8-15)13-14-12(18)16-9-5-2-6-10-16/h1-10H2 |
InChIキー |
OQJBFFCUFALWQL-UHFFFAOYSA-N |
SMILES |
C1CCN(CC1)C(=O)N=NC(=O)N2CCCCC2 |
異性体SMILES |
C1CCN(CC1)C(=O)/N=N/C(=O)N2CCCCC2 |
正規SMILES |
C1CCN(CC1)C(=O)N=NC(=O)N2CCCCC2 |
Key on ui other cas no. |
10465-81-3 |
ピクトグラム |
Irritant |
同義語 |
diazenedicarboxylic acid bis(N,N-piperidide) SR 4077 SR-4077 |
製品の起源 |
United States |
Retrosynthesis Analysis
AI-Powered Synthesis Planning: Our tool employs the Template_relevance Pistachio, Template_relevance Bkms_metabolic, Template_relevance Pistachio_ringbreaker, Template_relevance Reaxys, Template_relevance Reaxys_biocatalysis model, leveraging a vast database of chemical reactions to predict feasible synthetic routes.
One-Step Synthesis Focus: Specifically designed for one-step synthesis, it provides concise and direct routes for your target compounds, streamlining the synthesis process.
Accurate Predictions: Utilizing the extensive PISTACHIO, BKMS_METABOLIC, PISTACHIO_RINGBREAKER, REAXYS, REAXYS_BIOCATALYSIS database, our tool offers high-accuracy predictions, reflecting the latest in chemical research and data.
Strategy Settings
Precursor scoring | Relevance Heuristic |
---|---|
Min. plausibility | 0.01 |
Model | Template_relevance |
Template Set | Pistachio/Bkms_metabolic/Pistachio_ringbreaker/Reaxys/Reaxys_biocatalysis |
Top-N result to add to graph | 6 |
Feasible Synthetic Routes
試験管内研究製品の免責事項と情報
BenchChemで提示されるすべての記事および製品情報は、情報提供を目的としています。BenchChemで購入可能な製品は、生体外研究のために特別に設計されています。生体外研究は、ラテン語の "in glass" に由来し、生物体の外で行われる実験を指します。これらの製品は医薬品または薬として分類されておらず、FDAから任何の医療状態、病気、または疾患の予防、治療、または治癒のために承認されていません。これらの製品を人間または動物に体内に導入する形態は、法律により厳格に禁止されています。これらのガイドラインに従うことは、研究と実験において法的および倫理的な基準の遵守を確実にするために重要です。