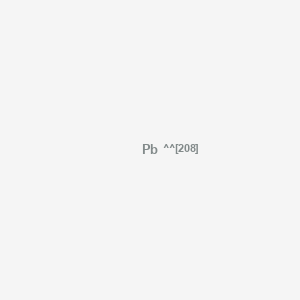
Lead-208
- 専門家チームからの見積もりを受け取るには、QUICK INQUIRYをクリックしてください。
- 品質商品を競争力のある価格で提供し、研究に集中できます。
説明
Lead-208 is the heaviest stable and non-radioactive isotope of the chemical element lead with the mass number 208 . It is also the heaviest known stable isotope of any element and has the heaviest known doubly magic atomic nucleus (closed nuclear shells) with Z = 82 (protons) and N = 126 (neutrons) .
Synthesis Analysis
In situ Pb isotope analyses of tiny melt inclusions using laser ablation–multi-collector–inductively coupled plasma–mass spectrometry (LA–MC–ICP–MS) are crucial for exploring the origins of mafic lavas . The described method improves the precision and accuracy of in situ Pb isotope analysis in low-Pb melt inclusions using LA–MC–ICP–MS .
Molecular Structure Analysis
The molecular formula of this compound is Pb . It has an isotopic mass of 207.976653 u . The nuclide mass is 207.9316746 u, which is the calculated nuclear mass without electrons . The nuclear binding energy is 1636.42913509 MeV per nucleus .
Chemical Reactions Analysis
This compound is suitable as a coolant and neutron moderator in nuclear reactors due to its particularly stable configuration of the nuclide . Its thermal neutron capture cross-section is very small (230 ± 12 μb) .
Physical and Chemical Properties Analysis
This compound has a mass number of 208, atomic number of 82, and contains 126 neutrons . It has a nuclear binding energy of 1636.42913509 MeV per nucleus . The charge radius is 5.5012 (13) femtometer fm .
科学的研究の応用
Precise Lead Isotope Analysis : 208Pb, along with other stable Pb isotopes, is used in precise isotope analysis by thermal ionization mass spectrometry (TIMS) and inductively coupled plasma mass spectrometry (ICP-MS). These techniques are crucial for understanding lead isotope compositions and their applications in geochemistry and environmental studies (Doucelance & Manhès, 2001).
Tracing Lead Metabolism and Therapeutic Treatment : 208Pb is used in stable lead isotope tracer studies for analyzing lead metabolism and therapeutic chelation treatment in animal models and humans. This involves enriching the natural abundance of one or more lead isotopes and analyzing them at sub-ppb levels (Woolard, Franks, & Smith, 1998).
Optimizing Isotopic Measurement Techniques : Research involving 208Pb focuses on optimizing techniques like double and triple spiking for high precision in lead isotopic measurements. This is important for improving the accuracy of lead isotope ratio determinations in various scientific applications (Galer, 1999).
Exploration and Ore Deposit Research : The isotopic composition of 208Pb in sulfide minerals and associated rocks is critical for understanding the source of metals and fluid pathways in ore deposits. It provides insights into the geological and chronological aspects of ore formation, aiding exploration and research in mineral deposits (Lambert & Ruíz, 1999).
Environmental Studies : Isotopic measurements of 208Pb are used to investigate anthropogenic lead in environments such as seawater and sediments. This helps in understanding the environmental dispersion of trace amounts of lead and its ecological impact (Munksgaard, Batterham, & Parry, 1998).
Nuclear Physics Research : In nuclear physics, 208Pb isotope measurements have implications for understanding proton-neutron interaction strengths, especially in contexts like the Schottky mass measurement of isotopes. This contributes to fundamental knowledge in nuclear physics and related fields (Chen et al., 2009).
作用機序
Safety and Hazards
特性
IUPAC Name |
lead-208 |
Source
|
---|---|---|
Source | PubChem | |
URL | https://pubchem.ncbi.nlm.nih.gov | |
Description | Data deposited in or computed by PubChem | |
InChI |
InChI=1S/Pb/i1+1 |
Source
|
Source | PubChem | |
URL | https://pubchem.ncbi.nlm.nih.gov | |
Description | Data deposited in or computed by PubChem | |
InChI Key |
WABPQHHGFIMREM-OUBTZVSYSA-N |
Source
|
Source | PubChem | |
URL | https://pubchem.ncbi.nlm.nih.gov | |
Description | Data deposited in or computed by PubChem | |
Canonical SMILES |
[Pb] |
Source
|
Source | PubChem | |
URL | https://pubchem.ncbi.nlm.nih.gov | |
Description | Data deposited in or computed by PubChem | |
Isomeric SMILES |
[208Pb] |
Source
|
Source | PubChem | |
URL | https://pubchem.ncbi.nlm.nih.gov | |
Description | Data deposited in or computed by PubChem | |
Molecular Formula |
Pb |
Source
|
Source | PubChem | |
URL | https://pubchem.ncbi.nlm.nih.gov | |
Description | Data deposited in or computed by PubChem | |
DSSTOX Substance ID |
DTXSID601047867 |
Source
|
Record name | Lead, isotope of mass 208 | |
Source | EPA DSSTox | |
URL | https://comptox.epa.gov/dashboard/DTXSID601047867 | |
Description | DSSTox provides a high quality public chemistry resource for supporting improved predictive toxicology. | |
Molecular Weight |
207.97665 g/mol |
Source
|
Source | PubChem | |
URL | https://pubchem.ncbi.nlm.nih.gov | |
Description | Data deposited in or computed by PubChem | |
CAS No. |
13966-28-4 |
Source
|
Record name | Lead, isotope of mass 208 | |
Source | ChemIDplus | |
URL | https://pubchem.ncbi.nlm.nih.gov/substance/?source=chemidplus&sourceid=0013966284 | |
Description | ChemIDplus is a free, web search system that provides access to the structure and nomenclature authority files used for the identification of chemical substances cited in National Library of Medicine (NLM) databases, including the TOXNET system. | |
Record name | Lead, isotope of mass 208 | |
Source | EPA DSSTox | |
URL | https://comptox.epa.gov/dashboard/DTXSID601047867 | |
Description | DSSTox provides a high quality public chemistry resource for supporting improved predictive toxicology. | |
試験管内研究製品の免責事項と情報
BenchChemで提示されるすべての記事および製品情報は、情報提供を目的としています。BenchChemで購入可能な製品は、生体外研究のために特別に設計されています。生体外研究は、ラテン語の "in glass" に由来し、生物体の外で行われる実験を指します。これらの製品は医薬品または薬として分類されておらず、FDAから任何の医療状態、病気、または疾患の予防、治療、または治癒のために承認されていません。これらの製品を人間または動物に体内に導入する形態は、法律により厳格に禁止されています。これらのガイドラインに従うことは、研究と実験において法的および倫理的な基準の遵守を確実にするために重要です。