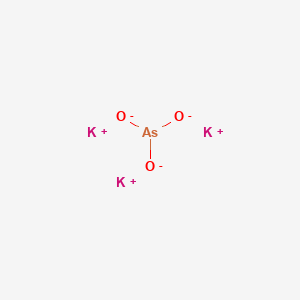
Tripotassium arsenite
- 専門家チームからの見積もりを受け取るには、QUICK INQUIRYをクリックしてください。
- 品質商品を競争力のある価格で提供し、研究に集中できます。
説明
Tripotassium arsenite is an inorganic compound with the chemical formula KAsO₂. It exists in two forms: potassium meta-arsenite (KAsO₂) and potassium ortho-arsenite (K₃AsO₃). Both forms contain arsenite ions (AsO₃³⁻ or AsO₂⁻) with arsenic in the +3 oxidation state. Historically, potassium arsenite was used in medicinal tonics, such as Fowler’s solution, but due to its high toxicity and carcinogenic nature, its use has been discontinued in medicine .
準備方法
Synthetic Routes and Reaction Conditions
Tripotassium arsenite can be prepared by heating arsenic trioxide (As₂O₃) with potassium hydroxide (KOH) in the presence of water. The reaction is as follows:
[ \text{As}_2\text{O}_3 (\text{aq}) + 2 \text{KOH} (\text{aq}) \rightarrow 2 \text{KAsO}_2 (\text{aq}) + \text{H}_2\text{O} ]
Industrial Production Methods
Industrial production of potassium arsenite involves similar methods but on a larger scale. The process requires precise control of reaction conditions to ensure the purity and concentration of the final product. The handling of arsenic compounds necessitates stringent safety measures due to their toxic nature .
化学反応の分析
Types of Reactions
Potassium arsenite undergoes various chemical reactions, including:
Oxidation: Potassium arsenite can be oxidized to form arsenate compounds.
Reduction: It can be reduced to elemental arsenic under certain conditions.
Substitution: Potassium arsenite can react with acids to yield toxic arsine gas (AsH₃).
Common Reagents and Conditions
Oxidation: Typically involves oxidizing agents such as potassium permanganate (KMnO₄) or hydrogen peroxide (H₂O₂).
Reduction: Reducing agents like zinc (Zn) or iron (Fe) can be used.
Substitution: Reactions with strong acids like hydrochloric acid (HCl) produce arsine gas.
Major Products
Oxidation: Forms potassium arsenate (K₃AsO₄).
Reduction: Produces elemental arsenic (As).
Substitution: Yields arsine gas (AsH₃) and other by-products.
科学的研究の応用
Potassium arsenite has several applications in scientific research:
Chemistry: Used in the synthesis of other arsenic compounds and as a reagent in various chemical reactions.
Biology: Studied for its effects on cellular processes and its role in enzyme inhibition.
Medicine: Historically used in treatments for conditions like anemia and psoriasis, though its use has been discontinued due to toxicity.
Industry: Employed as a rodenticide and in the production of certain glass and ceramics.
作用機序
The toxicity of potassium arsenite arises from arsenic’s high affinity for sulfhydryl groups. This affinity allows arsenic to form bonds with sulfur-containing enzymes, impairing their functionality. Key enzymes affected include glutathione reductase, glutathione peroxidases, thioredoxin reductase, and thioredoxin peroxidase. These interactions disrupt cellular redox balance and lead to oxidative stress, ultimately causing cell death .
類似化合物との比較
Similar Compounds
Potassium arsenate (K₃AsO₄): An inorganic compound used as a herbicide and pesticide.
Sodium arsenite (NaAsO₂): Another arsenic compound with similar uses and toxicity.
Uniqueness
Potassium arsenite is unique due to its historical use in medicine and its specific chemical properties, such as its ability to form different structures (meta-arsenite and ortho-arsenite). Its high toxicity and carcinogenic nature also distinguish it from other compounds .
特性
CAS番号 |
1332-10-1 |
---|---|
分子式 |
AsK3O3 |
分子量 |
240.215 g/mol |
IUPAC名 |
tripotassium;arsorite |
InChI |
InChI=1S/AsO3.3K/c2-1(3)4;;;/q-3;3*+1 |
InChIキー |
OZYUYLWHROWIFJ-UHFFFAOYSA-N |
SMILES |
[O-][As]([O-])[O-].[K+].[K+].[K+] |
正規SMILES |
[O-][As]([O-])[O-].[K+].[K+].[K+] |
Key on ui other cas no. |
36267-15-9 1332-10-1 |
同義語 |
Fowler's solution potassium arsenite solution |
製品の起源 |
United States |
試験管内研究製品の免責事項と情報
BenchChemで提示されるすべての記事および製品情報は、情報提供を目的としています。BenchChemで購入可能な製品は、生体外研究のために特別に設計されています。生体外研究は、ラテン語の "in glass" に由来し、生物体の外で行われる実験を指します。これらの製品は医薬品または薬として分類されておらず、FDAから任何の医療状態、病気、または疾患の予防、治療、または治癒のために承認されていません。これらの製品を人間または動物に体内に導入する形態は、法律により厳格に禁止されています。これらのガイドラインに従うことは、研究と実験において法的および倫理的な基準の遵守を確実にするために重要です。