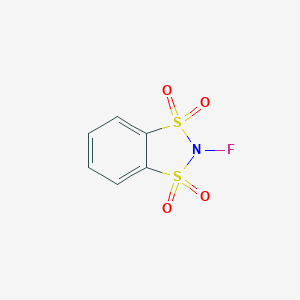
N-Fluoro-O-benzenedisulfonimide
概要
説明
N-Fluoro-O-benzenedisulfonimide is a versatile and widely used reagent in organic synthesis. It is known for its ability to introduce fluorine atoms into organic molecules, making it a valuable tool in the field of fluorine chemistry. This compound is particularly useful for electrophilic fluorination reactions, where it acts as a source of electrophilic fluorine.
準備方法
Synthetic Routes and Reaction Conditions
N-Fluoro-O-benzenedisulfonimide can be synthesized through several methods. One common approach involves the reaction of O-benzenedisulfonimide with a fluorinating agent such as elemental fluorine or a fluorine-containing compound. The reaction typically takes place in an organic solvent under controlled temperature and pressure conditions to ensure the desired product is obtained .
Industrial Production Methods
In industrial settings, the production of this compound often involves large-scale reactions using specialized equipment to handle the reactive and potentially hazardous fluorinating agents. The process is optimized for high yield and purity, with careful control of reaction parameters to minimize side reactions and by-products .
化学反応の分析
Types of Reactions
N-Fluoro-O-benzenedisulfonimide undergoes various types of chemical reactions, including:
Electrophilic Fluorination: It is commonly used to introduce fluorine atoms into aromatic and aliphatic compounds.
Amination: It can also act as an amination reagent, introducing amino groups into organic molecules.
Common Reagents and Conditions
The reactions involving this compound typically require the presence of a catalyst, such as palladium or other transition metals, to facilitate the reaction. Common solvents used include dichloromethane, acetonitrile, and dimethylformamide .
Major Products Formed
The major products formed from reactions with this compound include fluorinated aromatic and aliphatic compounds, as well as aminated derivatives. These products are valuable intermediates in the synthesis of pharmaceuticals, agrochemicals, and materials science .
科学的研究の応用
Medicinal Chemistry
N-Fluoro-O-benzenedisulfonimide plays a crucial role in the synthesis of fluorinated organic compounds that are vital in drug development. Fluorinated compounds often exhibit enhanced biological activity and metabolic stability, making them desirable in pharmaceuticals.
Case Study:
A study demonstrated the use of this compound in the selective fluorination of aromatic compounds. The resulting fluorinated products showed improved binding affinities in biological assays, highlighting their potential as drug candidates .
Materials Science
The compound is employed in the development of new materials with enhanced properties. Fluorination can significantly alter the physical and chemical properties of polymers and other materials, leading to improved performance in various applications.
Data Table: Properties of Fluorinated Materials
Material Type | Property Enhanced | Application Area |
---|---|---|
Polymers | Chemical resistance | Coatings and adhesives |
Organic compounds | Thermal stability | Electronics |
Agrochemicals | Bioactivity | Crop protection |
Biological Studies
Fluorinated compounds serve as useful probes in biological research, allowing scientists to investigate enzyme mechanisms and protein-ligand interactions. The unique properties of fluorine can enhance the visibility and tracking of these compounds in biological systems.
Example:
Research utilizing this compound has shown its effectiveness in labeling biomolecules for imaging studies, providing insights into cellular processes .
作用機序
The mechanism of action of N-Fluoro-O-benzenedisulfonimide involves the transfer of a fluorine atom to the target molecule. This process typically proceeds through an electrophilic substitution mechanism, where the fluorine atom replaces a hydrogen atom or another substituent on the target molecule. The presence of a catalyst, such as palladium, can facilitate this process by stabilizing the intermediate species and lowering the activation energy of the reaction .
類似化合物との比較
N-Fluoro-O-benzenedisulfonimide is part of a class of electrophilic fluorinating agents that includes compounds such as N-fluorobenzenesulfonimide, N-fluorocamphorsultam, and N-fluorobinaphthyldisulfonimide . Compared to these similar compounds, this compound offers unique advantages in terms of stability, ease of handling, and reactivity. It is less toxic and more stable than elemental fluorine, making it a safer and more practical choice for many applications .
List of Similar Compounds
- N-Fluorobenzenesulfonimide
- N-Fluorocamphorsultam
- N-Fluorobinaphthyldisulfonimide
This compound stands out due to its versatility and effectiveness in a wide range of chemical transformations, making it an invaluable reagent in both academic and industrial research.
生物活性
N-Fluoro-O-benzenedisulfonimide (NFOBS) is a fluorinating agent that has garnered attention in the field of medicinal chemistry due to its unique biological activities and applications. This compound, a stable and efficient electrophilic fluorination reagent, has been utilized in various synthetic processes, particularly in the development of pharmaceuticals and agrochemicals. This article explores the biological activity of NFOBS, supported by data tables, case studies, and detailed research findings.
NFOBS is synthesized from o-benzenedisulfonimide through direct fluorination methods. The presence of fluorine in organic compounds significantly alters their physicochemical properties, enhancing lipophilicity, metabolic stability, and bioavailability. The introduction of fluorine can also modify the acidity and basicity of molecules, which is crucial for their biological activity .
The mechanism by which NFOBS exerts its biological effects primarily involves its role as an electrophilic fluorination agent. This allows for the selective introduction of fluorine into various substrates, leading to the formation of biologically active compounds. The unique reactivity of NFOBS enables it to participate in diverse reactions, including nucleophilic substitutions and radical reactions, which are essential in drug development .
Antiviral and Anticancer Properties
Recent studies have demonstrated that NFOBS derivatives exhibit significant antiviral and anticancer activities. For instance, compounds synthesized using NFOBS have shown promising results against human cytomegalovirus (HCMV) and various cancer cell lines. In vitro assays indicated that certain fluoro-substituted derivatives achieved an IC50 value around 10 μM against HCMV and exhibited cytotoxicity against glioblastoma (GBM6) with IC50 values ranging from 36 to 80 μM .
Table 1: Biological Activity of NFOBS Derivatives
Compound | Target | IC50 (μM) | Activity Type |
---|---|---|---|
4d | HCMV | ~10 | Antiviral |
4l | GBM6 | 36-80 | Cytotoxicity |
5f | MDA MB231 | 36-80 | Cytotoxicity |
These findings suggest that the incorporation of fluorine into the structure significantly enhances the biological activity of these compounds compared to their non-fluorinated counterparts.
Mechanistic Insights
The mechanism underlying the anticancer activity involves apoptosis induction in cancer cells. Studies have shown that treatment with NFOBS derivatives leads to increased production of reactive oxygen species (ROS), which disrupt mitochondrial function and promote cell death through apoptosis . This highlights the potential of NFOBS as a lead compound in developing new anticancer therapies.
Case Study 1: Antiviral Activity Against HCMV
In a controlled study, researchers evaluated several NFOBS-derived compounds for their antiviral efficacy against HCMV. The study revealed that specific derivatives not only inhibited viral replication but also reduced cytopathic effects on infected cells. The most effective compounds were noted to have structural features conducive to enhanced interaction with viral components .
Case Study 2: Cytotoxicity in Glioblastoma Cells
Another significant study focused on the cytotoxic effects of NFOBS derivatives on glioblastoma cells. The results indicated a dose-dependent response where higher concentrations led to increased cell death rates. Flow cytometry analysis confirmed that treated cells exhibited characteristics typical of late-stage apoptosis, reinforcing the therapeutic potential of these compounds in cancer treatment .
特性
IUPAC Name |
2-fluoro-1λ6,3λ6,2-benzodithiazole 1,1,3,3-tetraoxide | |
---|---|---|
Source | PubChem | |
URL | https://pubchem.ncbi.nlm.nih.gov | |
Description | Data deposited in or computed by PubChem | |
InChI |
InChI=1S/C6H4FNO4S2/c7-8-13(9,10)5-3-1-2-4-6(5)14(8,11)12/h1-4H | |
Source | PubChem | |
URL | https://pubchem.ncbi.nlm.nih.gov | |
Description | Data deposited in or computed by PubChem | |
InChI Key |
ILXAULYORNMOAX-UHFFFAOYSA-N | |
Source | PubChem | |
URL | https://pubchem.ncbi.nlm.nih.gov | |
Description | Data deposited in or computed by PubChem | |
Canonical SMILES |
C1=CC=C2C(=C1)S(=O)(=O)N(S2(=O)=O)F | |
Source | PubChem | |
URL | https://pubchem.ncbi.nlm.nih.gov | |
Description | Data deposited in or computed by PubChem | |
Molecular Formula |
C6H4FNO4S2 | |
Source | PubChem | |
URL | https://pubchem.ncbi.nlm.nih.gov | |
Description | Data deposited in or computed by PubChem | |
DSSTOX Substance ID |
DTXSID20565505 | |
Record name | 2-Fluoro-1H-1lambda~6~,3lambda~6~,2-benzodithiazole-1,1,3,3(2H)-tetrone | |
Source | EPA DSSTox | |
URL | https://comptox.epa.gov/dashboard/DTXSID20565505 | |
Description | DSSTox provides a high quality public chemistry resource for supporting improved predictive toxicology. | |
Molecular Weight |
237.2 g/mol | |
Source | PubChem | |
URL | https://pubchem.ncbi.nlm.nih.gov | |
Description | Data deposited in or computed by PubChem | |
CAS No. |
1344-80-5 | |
Record name | 2-Fluoro-1H-1lambda~6~,3lambda~6~,2-benzodithiazole-1,1,3,3(2H)-tetrone | |
Source | EPA DSSTox | |
URL | https://comptox.epa.gov/dashboard/DTXSID20565505 | |
Description | DSSTox provides a high quality public chemistry resource for supporting improved predictive toxicology. | |
Retrosynthesis Analysis
AI-Powered Synthesis Planning: Our tool employs the Template_relevance Pistachio, Template_relevance Bkms_metabolic, Template_relevance Pistachio_ringbreaker, Template_relevance Reaxys, Template_relevance Reaxys_biocatalysis model, leveraging a vast database of chemical reactions to predict feasible synthetic routes.
One-Step Synthesis Focus: Specifically designed for one-step synthesis, it provides concise and direct routes for your target compounds, streamlining the synthesis process.
Accurate Predictions: Utilizing the extensive PISTACHIO, BKMS_METABOLIC, PISTACHIO_RINGBREAKER, REAXYS, REAXYS_BIOCATALYSIS database, our tool offers high-accuracy predictions, reflecting the latest in chemical research and data.
Strategy Settings
Precursor scoring | Relevance Heuristic |
---|---|
Min. plausibility | 0.01 |
Model | Template_relevance |
Template Set | Pistachio/Bkms_metabolic/Pistachio_ringbreaker/Reaxys/Reaxys_biocatalysis |
Top-N result to add to graph | 6 |
Feasible Synthetic Routes
試験管内研究製品の免責事項と情報
BenchChemで提示されるすべての記事および製品情報は、情報提供を目的としています。BenchChemで購入可能な製品は、生体外研究のために特別に設計されています。生体外研究は、ラテン語の "in glass" に由来し、生物体の外で行われる実験を指します。これらの製品は医薬品または薬として分類されておらず、FDAから任何の医療状態、病気、または疾患の予防、治療、または治癒のために承認されていません。これらの製品を人間または動物に体内に導入する形態は、法律により厳格に禁止されています。これらのガイドラインに従うことは、研究と実験において法的および倫理的な基準の遵守を確実にするために重要です。