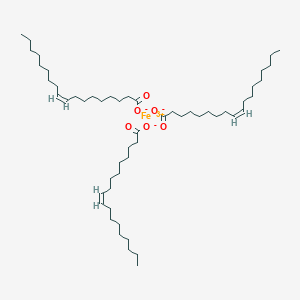
Ferric oleate
説明
Ferric oleate is a compound that has been used in various scientific and industrial applications . It is often used as a chemical reagent and as an ingredient of heavy-metal soaps, which are used in lubricating greases, gel thickeners, and in paints as driers and flatting agents .
Synthesis Analysis
Ferric oleate can be synthesized and applied as a catalyst in the aquathermolysis reaction of heavy crude oil . It has been found to be more efficient for heavy oil cracking than Co and Ni oleates .Chemical Reactions Analysis
Ferric oleate has been used in the aquathermolysis reaction of Shengli heavy oil . It was found to be more efficient for heavy oil cracking than Co and Ni oleates . It was superior to oleic acid and inorganic ferric nitrate and achieved the highest viscosity reduction rate of up to 86.1% .Physical And Chemical Properties Analysis
Ferric oleate is soluble in alcohol, ether, and acids. It is insoluble in water . More detailed physical and chemical properties are not available in the search results.科学的研究の応用
Aquathermolysis of Heavy Crude Oil
Ferric oleate has been synthesized and applied as a catalyst in the aquathermolysis reaction of Shengli heavy oil . It was found to be more efficient for heavy oil cracking than Co and Ni oleates . Besides, it was superior to oleic acid and inorganic ferric nitrate and achieved the highest viscosity reduction rate of up to 86.1% .
Viscosity Reduction in Heavy Crude Oil
Ferric oleate contributes to a significant increase in the content of light components and decrease in the content of resin, N and S . The as-prepared ferric oleate showed good activity for reducing the viscosity and improving the quality of the heavy crude oil .
Catalytic Oxidation Modification of Heavy Oil
Ferric oleate was utilized in a study on the catalytic oxidation modification effect of heavy oil at low temperature . The catalytic oxidation system consists of a catalyst, an oxidant, and a proton donor . Four different complexes of iron-based catalysts were utilized: ferric oleate, iron naphthenate, EDTA–FeNa, and EDDHA–FeNa . Catalytic oxidation processes with these catalysts produced four types of oxygenated oil .
Low-Temperature Catalytic Oxidation of Heavy Oil
This work explores the low-temperature catalytic oxidation of heavy oil (140 °C), resulting in structural changes with reduced heavy components and increased light components . The results show that EDDHA–FeNa is more favorable for the catalytic oxidation of heavy oil in a low-temperature environment, achieving a viscosity reduction rate of 78.57% .
作用機序
- The role of Ferric oleate is to reduce the viscosity of heavy crude oil, thereby improving its fluidity and facilitating extraction .
- The compound achieves this by breaking down complex hydrocarbon molecules into lighter components, enhancing their mobility and flow .
- However, possible mechanisms include hydrogenation, pyrolysis, depolymerization, isomerization, ring-opening, esterification, reconstruction, and oxygenation .
Target of Action
Mode of Action
Biochemical Pathways
Pharmacokinetics
Result of Action
Action Environment
Safety and Hazards
特性
IUPAC Name |
iron(3+);(Z)-octadec-9-enoate | |
---|---|---|
Source | PubChem | |
URL | https://pubchem.ncbi.nlm.nih.gov | |
Description | Data deposited in or computed by PubChem | |
InChI |
InChI=1S/3C18H34O2.Fe/c3*1-2-3-4-5-6-7-8-9-10-11-12-13-14-15-16-17-18(19)20;/h3*9-10H,2-8,11-17H2,1H3,(H,19,20);/q;;;+3/p-3/b3*10-9-; | |
Source | PubChem | |
URL | https://pubchem.ncbi.nlm.nih.gov | |
Description | Data deposited in or computed by PubChem | |
InChI Key |
HOIQWTMREPWSJY-GNOQXXQHSA-K | |
Source | PubChem | |
URL | https://pubchem.ncbi.nlm.nih.gov | |
Description | Data deposited in or computed by PubChem | |
Canonical SMILES |
CCCCCCCCC=CCCCCCCCC(=O)[O-].CCCCCCCCC=CCCCCCCCC(=O)[O-].CCCCCCCCC=CCCCCCCCC(=O)[O-].[Fe+3] | |
Source | PubChem | |
URL | https://pubchem.ncbi.nlm.nih.gov | |
Description | Data deposited in or computed by PubChem | |
Isomeric SMILES |
CCCCCCCC/C=C\CCCCCCCC(=O)[O-].CCCCCCCC/C=C\CCCCCCCC(=O)[O-].CCCCCCCC/C=C\CCCCCCCC(=O)[O-].[Fe+3] | |
Source | PubChem | |
URL | https://pubchem.ncbi.nlm.nih.gov | |
Description | Data deposited in or computed by PubChem | |
Molecular Formula |
C54H99FeO6 | |
Source | PubChem | |
URL | https://pubchem.ncbi.nlm.nih.gov | |
Description | Data deposited in or computed by PubChem | |
Molecular Weight |
900.2 g/mol | |
Source | PubChem | |
URL | https://pubchem.ncbi.nlm.nih.gov | |
Description | Data deposited in or computed by PubChem | |
Product Name |
Ferric oleate | |
CAS RN |
1120-45-2 | |
Record name | Ferric oleate | |
Source | ChemIDplus | |
URL | https://pubchem.ncbi.nlm.nih.gov/substance/?source=chemidplus&sourceid=0001120452 | |
Description | ChemIDplus is a free, web search system that provides access to the structure and nomenclature authority files used for the identification of chemical substances cited in National Library of Medicine (NLM) databases, including the TOXNET system. | |
Record name | Iron trioleate | |
Source | European Chemicals Agency (ECHA) | |
URL | https://echa.europa.eu/substance-information/-/substanceinfo/100.013.009 | |
Description | The European Chemicals Agency (ECHA) is an agency of the European Union which is the driving force among regulatory authorities in implementing the EU's groundbreaking chemicals legislation for the benefit of human health and the environment as well as for innovation and competitiveness. | |
Explanation | Use of the information, documents and data from the ECHA website is subject to the terms and conditions of this Legal Notice, and subject to other binding limitations provided for under applicable law, the information, documents and data made available on the ECHA website may be reproduced, distributed and/or used, totally or in part, for non-commercial purposes provided that ECHA is acknowledged as the source: "Source: European Chemicals Agency, http://echa.europa.eu/". Such acknowledgement must be included in each copy of the material. ECHA permits and encourages organisations and individuals to create links to the ECHA website under the following cumulative conditions: Links can only be made to webpages that provide a link to the Legal Notice page. | |
Record name | FERRIC OLEATE | |
Source | FDA Global Substance Registration System (GSRS) | |
URL | https://gsrs.ncats.nih.gov/ginas/app/beta/substances/C1NE1661VN | |
Description | The FDA Global Substance Registration System (GSRS) enables the efficient and accurate exchange of information on what substances are in regulated products. Instead of relying on names, which vary across regulatory domains, countries, and regions, the GSRS knowledge base makes it possible for substances to be defined by standardized, scientific descriptions. | |
Explanation | Unless otherwise noted, the contents of the FDA website (www.fda.gov), both text and graphics, are not copyrighted. They are in the public domain and may be republished, reprinted and otherwise used freely by anyone without the need to obtain permission from FDA. Credit to the U.S. Food and Drug Administration as the source is appreciated but not required. | |
Retrosynthesis Analysis
AI-Powered Synthesis Planning: Our tool employs the Template_relevance Pistachio, Template_relevance Bkms_metabolic, Template_relevance Pistachio_ringbreaker, Template_relevance Reaxys, Template_relevance Reaxys_biocatalysis model, leveraging a vast database of chemical reactions to predict feasible synthetic routes.
One-Step Synthesis Focus: Specifically designed for one-step synthesis, it provides concise and direct routes for your target compounds, streamlining the synthesis process.
Accurate Predictions: Utilizing the extensive PISTACHIO, BKMS_METABOLIC, PISTACHIO_RINGBREAKER, REAXYS, REAXYS_BIOCATALYSIS database, our tool offers high-accuracy predictions, reflecting the latest in chemical research and data.
Strategy Settings
Precursor scoring | Relevance Heuristic |
---|---|
Min. plausibility | 0.01 |
Model | Template_relevance |
Template Set | Pistachio/Bkms_metabolic/Pistachio_ringbreaker/Reaxys/Reaxys_biocatalysis |
Top-N result to add to graph | 6 |
Feasible Synthetic Routes
試験管内研究製品の免責事項と情報
BenchChemで提示されるすべての記事および製品情報は、情報提供を目的としています。BenchChemで購入可能な製品は、生体外研究のために特別に設計されています。生体外研究は、ラテン語の "in glass" に由来し、生物体の外で行われる実験を指します。これらの製品は医薬品または薬として分類されておらず、FDAから任何の医療状態、病気、または疾患の予防、治療、または治癒のために承認されていません。これらの製品を人間または動物に体内に導入する形態は、法律により厳格に禁止されています。これらのガイドラインに従うことは、研究と実験において法的および倫理的な基準の遵守を確実にするために重要です。