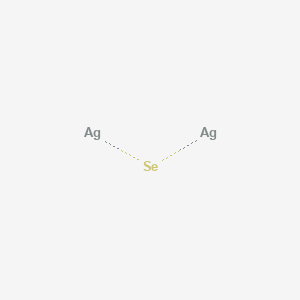
Silver selenide (Ag2Se)
説明
Silver selenide (Ag₂Se) is a I-VI semiconductor with an orthorhombic crystal structure (naumannite) and a tunable optical bandgap of 1.2–1.8 eV . It exhibits two stable phases: α-Ag₂Se (low-temperature orthorhombic) and β-Ag₂Se (high-temperature cubic), with the latter being pivotal for thermoelectric applications due to its superionic conductivity . Ag₂Se is synthesized via diverse methods, including green chemistry routes using fructose and starch , mechanical alloying , and pulsed laser deposition (PLD) . Its applications span thermoelectrics, optoelectronics, biomedical imaging, and magnetoresistive devices .
準備方法
Synthetic Routes and Reaction Conditions: Silver selenide (Ag2Se) can be synthesized through various methods, including:
Electrodeposition: This method involves the reduction of silver ions in the presence of selenide ions.
Chemical Bath Deposition: This involves the reaction of silver nitrate with sodium selenide in an aqueous solution.
Vacuum Evaporation: Silver selenide (Ag2Se) alloy is evaporated in a vacuum and then annealed to form thin films.
Industrial Production Methods: Industrial production of silver selenide often employs scalable methods such as electrodeposition, which allows for the fabrication of large-area films. This method is cost-effective and suitable for industrial applications .
Types of Reactions:
Reduction: Silver selenide (Ag2Se) can be reduced back to elemental silver and selenium under certain conditions.
Substitution: Silver selenide (Ag2Se) can participate in substitution reactions where selenium is replaced by other chalcogens.
Common Reagents and Conditions:
Oxidizing Agents: Oxygen and sulfur dioxide are commonly used in the oxidation of silver selenide.
Reducing Agents: Hydrogen or other reducing gases can be used to reduce silver selenide.
Major Products Formed:
Silver Selenite (Ag₂SeO₃): Formed during the oxidation of silver selenide.
Elemental Silver and Selenium: Formed during the reduction of silver selenide.
科学的研究の応用
Thermoelectric Applications
Ag₂Se is recognized for its excellent thermoelectric properties, making it a promising candidate for energy conversion technologies. It has been studied as an alternative to traditional thermoelectric materials like bismuth telluride (Bi₂Te₃) and lead selenide (PbSe), which are often toxic or expensive.
Performance Metrics
- Power Factor (PF) : Recent studies have reported an average PF of 617 mW/m²K² for Ag₂Se thin films, with maximum values reaching up to 825 mW/m²K² at room temperature .
- Figure of Merit (ZT) : The ZT value can reach up to 0.46, indicating good efficiency for thermoelectric applications .
Case Study: Rb-Doped Ag₂Se
Research has shown that doping Ag₂Se with rubidium can enhance its thermoelectric performance. The introduction of rubidium leads to a band gap increase and improved Seebeck coefficient, resulting in a ZT value ranging from 0.6 to 1.0 between 300 K and 1000 K .
Optoelectronic Applications
Ag₂Se exhibits remarkable optoelectronic properties, making it suitable for applications in photodetectors and solar cells.
Nanoparticle Synthesis
A green synthesis method using fructose as a reducing agent has been developed to produce Ag₂Se nanoparticles (NPs). These NPs demonstrate excellent optical properties and stability, which are crucial for their use in optoelectronic devices .
Optical Properties
- The synthesized Ag₂Se NPs show significant absorption across the visible spectrum, making them suitable for light-harvesting applications in photovoltaic devices .
Biomedical Applications
The biocompatibility and antibacterial properties of Ag₂Se nanoparticles have opened avenues for their use in medical applications.
Antibacterial Activity
Studies have demonstrated that Ag₂Se NPs exhibit bactericidal effects against various pathogens, making them potential candidates for antimicrobial coatings and therapeutic agents .
Cytotoxicity Studies
In vitro cytotoxicity assessments indicate that these nanoparticles can be safely used in biomedical applications without significant toxicity to human cells .
Industrial Applications
Ag₂Se is also utilized in various industrial sectors, particularly in infrared detection and imaging technologies.
Infrared Sensors
Due to its semiconductor properties, Ag₂Se is used in infrared detectors, where it plays a crucial role in the detection of thermal radiation .
Summary Table of Applications
Application Area | Key Properties | Performance Metrics |
---|---|---|
Thermoelectric | High ZT values | PF: up to 825 mW/m²K² |
Optoelectronic | Strong optical absorption | Effective light harvesting |
Biomedical | Antibacterial activity | Low cytotoxicity |
Industrial | Infrared detection | Reliable sensor performance |
作用機序
The mechanism of action of silver selenide in thermoelectric applications involves the conversion of thermal energy into electrical energy through the Seebeck effect. The material’s low thermal conductivity and high electrical conductivity make it an efficient thermoelectric material . In oxidation reactions, silver selenide forms silver selenite, which involves the transfer of electrons and the formation of new chemical bonds .
類似化合物との比較
Thermoelectric Performance: Ag₂Se vs. Bi₂Te₃
Ag₂Se has emerged as a sustainable alternative to n-type Bi₂Te₃, the benchmark room-temperature thermoelectric material. Key comparisons include:
Key Insights :
- Ag₂Se’s low thermal conductivity and high electrical conductivity enable superior thermoelectric efficiency at room temperature .
- Bi₂Te₃ suffers from Te scarcity and brittleness, whereas Ag₂Se-based flexible films (e.g., on nylon membranes) demonstrate durability in wearable devices .
Key Insights :
- Cu₂Se degrades at elevated temperatures due to selenium evaporation, limiting its high-temperature utility .
- Ag₂Se synthesized via ball milling shows lower thermal stability than Cu₂Se due to porosity but excels in room-temperature applications .
Electronic and Optical Properties: Ag₂Se vs. SnSe
SnSe, a high-ZT (2.6 at 923 K) p-type material, contrasts with n-type Ag₂Se in electronic structure:
Key Insights :
- SnSe outperforms Ag₂Se at high temperatures but requires complex doping for n-type behavior .
- Ag₂Se’s tunable bandgap facilitates optoelectronic applications, such as near-infrared sensors .
Toxicity and Biocompatibility: Ag₂Se vs. Other Selenides
Ag₂Se nanoparticles (NPs) exhibit lower toxicity compared to pure Ag or Se NPs when encapsulated in polymer matrices like arabinogalactan (AG):
Property | Ag₂Se-AG Nanocomposite | Ag NPs | Se NPs | |
---|---|---|---|---|
Cytotoxicity | Low (IC₅₀ > 100 µg/mL) | High (IC₅₀ ~10 µg/mL) | Moderate | |
Bactericidal Effect | Moderate | High | Low |
Key Insights :
- Encapsulation reduces Ag₂Se’s neurotoxicity, making it viable for biomedical applications like fluorescent labeling .
生物活性
Silver selenide (Ag2Se) is a compound that has garnered significant attention in the fields of nanotechnology and biomedicine due to its unique properties and biological activities. This article provides a comprehensive overview of the biological activity of Ag2Se, focusing on its antimicrobial, antioxidant, and cytotoxic effects, as well as its potential applications in biomedical fields.
Silver selenide is a semiconductor compound formed by silver and selenium. It is primarily synthesized through various methods, including chemical reduction and green synthesis techniques. Recent studies have highlighted the effectiveness of using natural extracts for the biosynthesis of Ag2Se nanoparticles (NPs), which not only simplifies the process but also enhances the biocompatibility of the resulting nanoparticles.
Synthesis Methods
- Green Synthesis : Utilizing plant extracts such as Melilotus officinalis for the synthesis of Ag2Se NPs has shown promising results in terms of antimicrobial activity and biocompatibility .
- Chemical Reduction : Traditional methods involve the reduction of silver nitrate with selenium sources under controlled conditions, often using stabilizing agents like starch or fructose .
Biological Activities
The biological activities of Ag2Se have been extensively studied, revealing several beneficial properties:
1. Antimicrobial Activity
Ag2Se exhibits significant antimicrobial properties against various pathogens, including antibiotic-resistant strains. The Minimum Inhibitory Concentration (MIC) and Minimum Bactericidal Concentration (MBC) values have been determined for specific bacteria:
Bacteria | MIC (µg/ml) | MBC (µg/ml) |
---|---|---|
Pseudomonas aeruginosa | 3.12 | 50 |
Escherichia coli | 5.0 | 20 |
These findings suggest that Ag2Se NPs can effectively inhibit bacterial growth, making them potential candidates for antimicrobial applications in medicine .
2. Antioxidant Activity
The antioxidant capacity of Ag2Se NPs has been evaluated using various assays. For instance, the DPPH scavenging assay yielded an IC50 value of 58.52 µg/ml, indicating moderate antioxidant activity . This property is crucial for protecting cells from oxidative stress, which is linked to numerous diseases.
3. Cytotoxicity
Cytotoxic studies have shown that Ag2Se NPs can induce cell death in cancer cell lines while exhibiting low toxicity towards normal cells. This selective cytotoxicity is essential for developing targeted cancer therapies .
Case Studies
Several studies have explored the biological effects of Ag2Se in vivo and in vitro:
- In Vivo Toxicity Studies : Research involving white male rats demonstrated that subacute exposure to Ag2Se at a dose of 500 mg/kg did not result in significant histopathological changes in brain tissue or other organs, indicating low acute toxicity . However, some alterations were noted in neuronal health, suggesting further investigation into long-term effects is necessary.
- Antibiofilm Activity : A study assessed the antibiofilm efficacy of Ag2Se against Pseudomonas aeruginosa. The results indicated that sub-MIC concentrations significantly reduced biofilm formation, highlighting its potential use in preventing biofilm-associated infections .
化学反応の分析
Aqueous Precipitation
Reaction of Ag⁺ with Se²⁻ precursors under alkaline conditions:Starch acts as a stabilizer, forming nanoparticles (4–16 nm) via hydroxyl group coordination .
Cation Exchange
Room-temperature transformation of Cu₂Se to Ag₂Se via ion exchange:Thermodynamic driving force: ΔG ≈ −210 kJ/mol .
Surface Etching and Vapor Interaction
Ag reacts with Se vapor to form Ag₂Se nanoparticles (Fig. S2, ):
- Reaction rate: Ag₂Te > Ag₂Se > Ag₂S .
- Se vapor diffusion enables nanoparticle growth on Ag surfaces .
Kinetic Control via Stabilizers
Starch and arabinogalactan (AG) modulate reaction rates by:
- Coordinating Ag⁺ ions via hydroxyl groups .
- Passivating nanoparticle surfaces to prevent aggregation .
Phase Transitions
Ag₂Se exhibits structural polymorphism under varying conditions:
Phase | Structure | Stability Range | Band Gap (eV) | Conductivity (S/cm) |
---|---|---|---|---|
β-Ag₂Se | Orthorhombic | < 130°C | 0.02–0.22 | 10⁻⁴ |
α-Ag₂Se | Cubic | > 130°C | – | ~2 |
Metastable | Monoclinic | Nanoscale (colloidal) | 0.15 | – |
Oxidation Reactions
Ag₂Se oxidizes in O₂-rich environments:
Thermoelectric Films
Selenization optimizes carrier concentration and lattice thermal conductivity:
Selenization Time (min) | Ag Content (at.%) | σ (S/cm) | S (μV/K) |
---|---|---|---|
45 | 2.22 | 1.2×10⁵ | −52 |
60 | 2.19 | 8.5×10⁴ | −68 |
Biomedical Functionalization
Ag₂Se-AG nanocomposites (4% w/w Ag₂Se) show reduced toxicity due to Se’s protective effects .
Q & A
Basic Research Questions
Q. What are the primary synthesis methods for silver selenide (Ag₂Se) nanostructures, and how do experimental parameters influence crystallinity and phase purity?
Silver selenide can be synthesized via electrodeposition, template-engaged methods, or chemical conversion. For instance, cyclic voltammetry in acidic solutions (e.g., 0.2 M H₂SO₄ with AgNO₃ and H₂SeO₃) enables controlled electrodeposition by adjusting reduction potentials to stabilize Ag₂Se phases . Template methods using trigonal Se nanotubes yield crystalline Ag₂Se nanotubes, with morphology confirmed via SEM and XRD . Key parameters include pH (via Pourbaix diagrams), precursor concentrations, and deposition time, which directly affect phase composition (e.g., orthorhombic vs. monoclinic phases) .
Q. How do thermoelectric properties of Ag₂Se vary with temperature and doping, and what experimental setups are used to measure them?
Ag₂Se exhibits a high Seebeck coefficient (~200 µV/K) and low thermal conductivity (~1 W/m·K) at room temperature. Resistivity and carrier mobility are measured using four-probe techniques, while doping with elements like Te or Sb alters carrier concentration and thermoelectric efficiency. For example, 1% Te doping increases power factor by 30% due to optimized carrier scattering . Temperature-dependent hysteresis in resistivity (observed between heating/cooling cycles) requires controlled-environment chambers to minimize thermal drift .
Q. What are the solubility characteristics of Ag₂Se in aqueous systems, and how do pH and ionic strength affect speciation?
Under nitrogen atmosphere at 25°C, Ag₂Se forms two aqueous species: [Ag₂(HSe)(OH)] in acidic media (pβ = 48.49) and [Ag(Se)₂(OH)]⁴⁻ in alkaline conditions (pβ = 24.07). Stability constants are calculated via potentiometric titrations at fixed ionic strength (1 M), with speciation confirmed by mass-balance equations and solubility experiments .
Advanced Research Questions
Q. How can contradictions in thermoelectric data (e.g., Seebeck coefficient vs. conductivity) be resolved in Ag₂Se thin films?
Discrepancies often arise from inhomogeneous doping or phase segregation. Advanced characterization techniques, such as EDX mapping and temperature-dependent Hall effect measurements, identify localized dopant distribution. For example, Ag₂Se films electrodeposited at −0.6 V vs. Ag/AgCl show 5% Se excess, reducing carrier mobility by 15% compared to stoichiometric films . Statistical error analysis (e.g., Monte Carlo simulations) quantifies uncertainties in thermoelectric parameter correlations .
Q. What methodological strategies mitigate hysteresis in Ag₂Se phase transformations during electrical measurements?
Hysteresis arises from first-order phase transitions (e.g., α ↔ β-Ag₂Se at ~130°C). To minimize this, use slow heating/cooling rates (<1°C/min) and pre-anneal samples at 150°C for 2 hours to stabilize phase boundaries. In-situ XRD during thermal cycling confirms reversible structural changes, while lock-in amplifier techniques improve signal-to-noise ratios in resistivity measurements .
Q. How do ionic and electronic conduction pathways in Ag₂Se-based composites (e.g., Ag₂Se/β-CD/graphene) influence electrochemical sensing performance?
In Ag₂Se/β-CD/graphene hybrids, β-CD enhances analyte adsorption via host-guest interactions, while graphene provides electronic conduction pathways. Electrochemical impedance spectroscopy (EIS) reveals a 50% reduction in charge-transfer resistance compared to pure Ag₂Se, enabling ultrasensitive detection of azithromycin (LOD = 0.1 nM) . Controlled defect engineering (e.g., Se vacancies) optimizes mixed ionic-electronic conductivity .
Q. What experimental evidence supports non-random mixing of Cd/Ag in ternary selenide glasses, and how does this affect Ag⁺ ion mobility?
EXAFS and high-energy XRD show Cd²⁺ ions occupy interstitial sites in Ag⁺-conducting pathways, blocking ion transport. In CdTe-AgI-As₂Se₃ glasses, 20 mol% CdTe reduces Ag⁺ conductivity by 90% due to Cd²⁺-induced site blocking. Haven ratio analysis (>1.5) confirms correlated Ag⁺ hopping, validated via tracer diffusion experiments with ¹¹⁰mAg .
Q. Methodological Guidance
Q. How to design reproducible electrodeposition protocols for Ag₂Se films using Pourbaix diagrams?
- Step 1 : Generate a Pourbaix diagram for Ag-Se-H₂O at 25°C to identify stable Ag₂Se regions (pH 0–14, 0 V vs. NHE) .
- Step 2 : Optimize electrolyte composition (e.g., 5 mM AgNO₃ + 5 mM H₂SeO₃ in 0.2 M H₂SO₄) and scan rate (10 mV/s) via cyclic voltammetry .
- Step 3 : Validate film stoichiometry using EDX and cross-reference with XRD to confirm phase purity .
Q. How to analyze conflicting data on Ag₂Se’s environmental stability in composite materials?
- Compare accelerated aging tests (e.g., 85°C/85% RH for 72 hours) with real-time degradation studies.
- Use XPS to track Se oxidation states: Se²⁻ → Se⁰ in humid conditions reduces shielding efficiency by 40% .
- Apply life-cycle assessment (LCA) frameworks to quantify nanoparticle release risks .
特性
InChI |
InChI=1S/2Ag.Se | |
---|---|---|
Source | PubChem | |
URL | https://pubchem.ncbi.nlm.nih.gov | |
Description | Data deposited in or computed by PubChem | |
InChI Key |
GNWCVDGUVZRYLC-UHFFFAOYSA-N | |
Source | PubChem | |
URL | https://pubchem.ncbi.nlm.nih.gov | |
Description | Data deposited in or computed by PubChem | |
Canonical SMILES |
[Se].[Ag].[Ag] | |
Source | PubChem | |
URL | https://pubchem.ncbi.nlm.nih.gov | |
Description | Data deposited in or computed by PubChem | |
Molecular Formula |
Ag2Se | |
Record name | silver selenide | |
Source | Wikipedia | |
URL | https://en.wikipedia.org/wiki/Silver_selenide | |
Description | Chemical information link to Wikipedia. | |
Source | PubChem | |
URL | https://pubchem.ncbi.nlm.nih.gov | |
Description | Data deposited in or computed by PubChem | |
DSSTOX Substance ID |
DTXSID2061644 | |
Record name | Silver selenide (Ag2Se) | |
Source | EPA DSSTox | |
URL | https://comptox.epa.gov/dashboard/DTXSID2061644 | |
Description | DSSTox provides a high quality public chemistry resource for supporting improved predictive toxicology. | |
Molecular Weight |
294.71 g/mol | |
Source | PubChem | |
URL | https://pubchem.ncbi.nlm.nih.gov | |
Description | Data deposited in or computed by PubChem | |
CAS No. |
1302-09-6 | |
Record name | Silver selenide (Ag2Se) | |
Source | CAS Common Chemistry | |
URL | https://commonchemistry.cas.org/detail?cas_rn=1302-09-6 | |
Description | CAS Common Chemistry is an open community resource for accessing chemical information. Nearly 500,000 chemical substances from CAS REGISTRY cover areas of community interest, including common and frequently regulated chemicals, and those relevant to high school and undergraduate chemistry classes. This chemical information, curated by our expert scientists, is provided in alignment with our mission as a division of the American Chemical Society. | |
Explanation | The data from CAS Common Chemistry is provided under a CC-BY-NC 4.0 license, unless otherwise stated. | |
Record name | Silver selenide | |
Source | ChemIDplus | |
URL | https://pubchem.ncbi.nlm.nih.gov/substance/?source=chemidplus&sourceid=0001302096 | |
Description | ChemIDplus is a free, web search system that provides access to the structure and nomenclature authority files used for the identification of chemical substances cited in National Library of Medicine (NLM) databases, including the TOXNET system. | |
Record name | Silver selenide (Ag2Se) | |
Source | EPA Chemicals under the TSCA | |
URL | https://www.epa.gov/chemicals-under-tsca | |
Description | EPA Chemicals under the Toxic Substances Control Act (TSCA) collection contains information on chemicals and their regulations under TSCA, including non-confidential content from the TSCA Chemical Substance Inventory and Chemical Data Reporting. | |
Record name | Silver selenide (Ag2Se) | |
Source | EPA DSSTox | |
URL | https://comptox.epa.gov/dashboard/DTXSID2061644 | |
Description | DSSTox provides a high quality public chemistry resource for supporting improved predictive toxicology. | |
Record name | Disilver selenide | |
Source | European Chemicals Agency (ECHA) | |
URL | https://echa.europa.eu/substance-information/-/substanceinfo/100.013.727 | |
Description | The European Chemicals Agency (ECHA) is an agency of the European Union which is the driving force among regulatory authorities in implementing the EU's groundbreaking chemicals legislation for the benefit of human health and the environment as well as for innovation and competitiveness. | |
Explanation | Use of the information, documents and data from the ECHA website is subject to the terms and conditions of this Legal Notice, and subject to other binding limitations provided for under applicable law, the information, documents and data made available on the ECHA website may be reproduced, distributed and/or used, totally or in part, for non-commercial purposes provided that ECHA is acknowledged as the source: "Source: European Chemicals Agency, http://echa.europa.eu/". Such acknowledgement must be included in each copy of the material. ECHA permits and encourages organisations and individuals to create links to the ECHA website under the following cumulative conditions: Links can only be made to webpages that provide a link to the Legal Notice page. | |
試験管内研究製品の免責事項と情報
BenchChemで提示されるすべての記事および製品情報は、情報提供を目的としています。BenchChemで購入可能な製品は、生体外研究のために特別に設計されています。生体外研究は、ラテン語の "in glass" に由来し、生物体の外で行われる実験を指します。これらの製品は医薬品または薬として分類されておらず、FDAから任何の医療状態、病気、または疾患の予防、治療、または治癒のために承認されていません。これらの製品を人間または動物に体内に導入する形態は、法律により厳格に禁止されています。これらのガイドラインに従うことは、研究と実験において法的および倫理的な基準の遵守を確実にするために重要です。