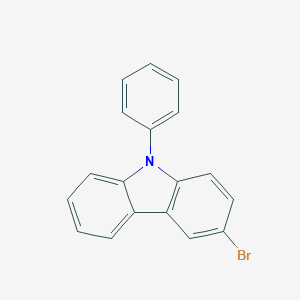
3-Bromo-9-phenylcarbazole
概要
説明
3-Bromo-9-phenylcarbazole is a heterocyclic organic compound used as an intermediate for synthesis . It is an off-white powder and is used in the manufacturing of OLED materials .
Synthesis Analysis
The synthesis of 3-Bromo-9-phenylcarbazole often involves strategic bromination of carbazole precursors. A specific method involves reacting 3-bromo-9H-carbazole (2.5 g, 0.010 mol) in bromobenzene (1.6 g, 0.010 mol), dibenzo-18-crown-6 (1.1 g, 0.0030 mol), copper (2) (1.2 g, 0.020 mol), into a 100 mL DMF to potassium acetate (2.8 g, 0.020 mol). The reaction is stirred for 4 hours at 120 degrees .
Molecular Structure Analysis
The molecular structure of 3-Bromo-9-phenylcarbazole derivatives has been elucidated through various spectroscopic and crystallographic techniques. The carbazole ring system is essentially planar and forms a dihedral angle with the phenyl ring, highlighting the compound’s rigid structure which is crucial for its electronic properties.
Chemical Reactions Analysis
Carbazole derivatives undergo various chemical reactions, including electrochemical polymerization and dimerization. These properties are fundamental for applications in electronic devices and materials science.
Physical And Chemical Properties Analysis
3-Bromo-9-phenylcarbazole is slightly soluble in water . It has a boiling point of 461.7±27.0 °C and a flash point of 233.0±23.7 °C .
科学的研究の応用
OLED Materials
3-Bromo-9-phenylcarbazole is used in the manufacturing of OLED materials . OLEDs (Organic Light Emitting Diodes) are used in various display and lighting technologies .
Pharmaceutical Intermediates
3-Bromo-9-phenylcarbazole is also used as an intermediate for pharmaceuticals . It can be used in the synthesis of various pharmaceutical compounds .
作用機序
Target of Action
3-Bromo-9-phenylcarbazole is a chemical compound used as an intermediate in synthesizing organic compounds . It is primarily used in the manufacturing of OLED materials . .
Mode of Action
The mode of action of 3-Bromo-9-phenylcarbazole is not well-studied. As an intermediate in the synthesis of organic compounds, it likely interacts with other molecules to form complex structures. In the context of OLED materials, these interactions could influence the electronic properties of the final product .
Biochemical Pathways
As an intermediate in organic synthesis, it is involved in various chemical reactions that lead to the formation of complex organic structures .
Pharmacokinetics
It is slightly soluble in water , which could influence its bioavailability and distribution.
Result of Action
As an intermediate in organic synthesis, its primary role is likely in the formation of complex organic structures .
Action Environment
Environmental factors can influence the action, efficacy, and stability of 3-Bromo-9-phenylcarbazole. For instance, it should be stored in a cool, dry place away from sources of heat or ignition . It is also recommended to avoid breathing its dust/fume/gas/mist/vapours/spray, indicating that its stability and action could be affected by exposure to air .
Safety and Hazards
3-Bromo-9-phenylcarbazole can cause skin irritation and serious eye irritation . It may also cause respiratory irritation . It is advised to avoid dust formation, breathing mist, gas or vapours, and contact with skin and eye . Use of personal protective equipment and adequate ventilation is recommended .
特性
IUPAC Name |
3-bromo-9-phenylcarbazole | |
---|---|---|
Source | PubChem | |
URL | https://pubchem.ncbi.nlm.nih.gov | |
Description | Data deposited in or computed by PubChem | |
InChI |
InChI=1S/C18H12BrN/c19-13-10-11-18-16(12-13)15-8-4-5-9-17(15)20(18)14-6-2-1-3-7-14/h1-12H | |
Source | PubChem | |
URL | https://pubchem.ncbi.nlm.nih.gov | |
Description | Data deposited in or computed by PubChem | |
InChI Key |
KUBSCXXKQGDPPD-UHFFFAOYSA-N | |
Source | PubChem | |
URL | https://pubchem.ncbi.nlm.nih.gov | |
Description | Data deposited in or computed by PubChem | |
Canonical SMILES |
C1=CC=C(C=C1)N2C3=C(C=C(C=C3)Br)C4=CC=CC=C42 | |
Source | PubChem | |
URL | https://pubchem.ncbi.nlm.nih.gov | |
Description | Data deposited in or computed by PubChem | |
Molecular Formula |
C18H12BrN | |
Source | PubChem | |
URL | https://pubchem.ncbi.nlm.nih.gov | |
Description | Data deposited in or computed by PubChem | |
DSSTOX Substance ID |
DTXSID70596708 | |
Record name | 3-Bromo-9-phenyl-9H-carbazole | |
Source | EPA DSSTox | |
URL | https://comptox.epa.gov/dashboard/DTXSID70596708 | |
Description | DSSTox provides a high quality public chemistry resource for supporting improved predictive toxicology. | |
Molecular Weight |
322.2 g/mol | |
Source | PubChem | |
URL | https://pubchem.ncbi.nlm.nih.gov | |
Description | Data deposited in or computed by PubChem | |
Product Name |
3-Bromo-9-phenylcarbazole | |
CAS RN |
1153-85-1 | |
Record name | 3-Bromo-9-phenyl-9H-carbazole | |
Source | EPA DSSTox | |
URL | https://comptox.epa.gov/dashboard/DTXSID70596708 | |
Description | DSSTox provides a high quality public chemistry resource for supporting improved predictive toxicology. | |
Record name | 3-Bromo-9-phenylcarbazole | |
Source | European Chemicals Agency (ECHA) | |
URL | https://echa.europa.eu/information-on-chemicals | |
Description | The European Chemicals Agency (ECHA) is an agency of the European Union which is the driving force among regulatory authorities in implementing the EU's groundbreaking chemicals legislation for the benefit of human health and the environment as well as for innovation and competitiveness. | |
Explanation | Use of the information, documents and data from the ECHA website is subject to the terms and conditions of this Legal Notice, and subject to other binding limitations provided for under applicable law, the information, documents and data made available on the ECHA website may be reproduced, distributed and/or used, totally or in part, for non-commercial purposes provided that ECHA is acknowledged as the source: "Source: European Chemicals Agency, http://echa.europa.eu/". Such acknowledgement must be included in each copy of the material. ECHA permits and encourages organisations and individuals to create links to the ECHA website under the following cumulative conditions: Links can only be made to webpages that provide a link to the Legal Notice page. | |
Synthesis routes and methods I
Procedure details
Synthesis routes and methods II
Procedure details
Synthesis routes and methods III
Procedure details
Synthesis routes and methods IV
Procedure details
Q & A
Q1: What are the main applications of 3-Bromo-9-phenylcarbazole?
A1: 3-Bromo-9-phenylcarbazole is not typically used directly in applications. Instead, it serves as a key intermediate in the synthesis of more complex molecules. [] These complex molecules, often incorporating the carbazole structure, are valuable in various fields, particularly as materials for OLEDs. []
Q2: What synthetic routes are commonly used to produce 3-Bromo-9-phenylcarbazole?
A2: The provided research paper summarizes and compares different synthetic routes for 3-Bromo-9-phenylcarbazole and its precursor, 9-phenylcarbazole. [] Researchers can compare these routes based on factors like yield, cost-effectiveness, and environmental impact to choose the most suitable method for their needs.
試験管内研究製品の免責事項と情報
BenchChemで提示されるすべての記事および製品情報は、情報提供を目的としています。BenchChemで購入可能な製品は、生体外研究のために特別に設計されています。生体外研究は、ラテン語の "in glass" に由来し、生物体の外で行われる実験を指します。これらの製品は医薬品または薬として分類されておらず、FDAから任何の医療状態、病気、または疾患の予防、治療、または治癒のために承認されていません。これらの製品を人間または動物に体内に導入する形態は、法律により厳格に禁止されています。これらのガイドラインに従うことは、研究と実験において法的および倫理的な基準の遵守を確実にするために重要です。