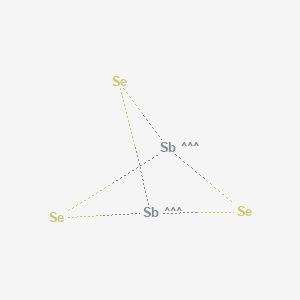
Antimony selenide (Sb2Se3)
説明
Antimony selenide (Sb2Se3) is an inorganic compound with the chemical formula Sb₂Se₃. It occurs naturally as the mineral antimonselite and crystallizes in an orthorhombic space group . In this compound, antimony has a formal oxidation state of +3, and selenium has an oxidation state of -2 . The compound is known for its black orthorhombic crystals with a metallic luster .
作用機序
Target of Action
Antimony triselenide (Sb2Se3) is an inorganic compound that primarily targets the semiconductor industry . It is a promising absorber material choice among inorganic semiconductors . The compound has a formal oxidation state of +3 for antimony and -2 for selenium .
Mode of Action
The mode of action of antimony triselenide is primarily through its semiconducting properties . It is used in the creation of thin-film solar cells . The compound may be formed by the reaction of antimony with selenium .
Biochemical Pathways
This process may govern the uptake and detoxification of both endogenous compounds and xenobiotics at the testis and brain blood barriers .
Pharmacokinetics
It is known that the compound has ahigh melting point of 611 °C , which may impact its bioavailability.
Result of Action
The result of antimony triselenide’s action is the creation of thin-film solar cells . The compound has been actively explored for this application, with a record light-to-electricity conversion efficiency of 9.2% reported .
Action Environment
The action of antimony triselenide can be influenced by environmental factors. For instance, the compound’s performance in solar cells can be enhanced by adjusting the S/S+Se atomic ratio . Additionally, the compound’s semiconducting properties, such as its band gap, can be affected by temperature .
生化学分析
Biochemical Properties
Selenium is involved in the conjugation of reduced glutathione to a wide number of exogenous and endogenous hydrophobic electrophiles .
Temporal Effects in Laboratory Settings
In laboratory settings, Antimony triselenide has been studied for its optical properties . It has been found that the morphological, optical, and structural properties of Antimony triselenide thin films are highly dependent on the amount of sulfur in the films .
準備方法
Antimony selenide (Sb2Se3) can be synthesized through various methods. One of the earliest methods involved treating an aqueous solution of potassium antimony tartrate with hydrogen selenide gas . Another method involves the direct reaction of antimony with selenium at high temperatures . Industrially, antimony triselenide thin films can be prepared using direct current magnetron sputtering followed by sulfurization in a quartz ampoule .
化学反応の分析
Antimony selenide (Sb2Se3) undergoes several types of chemical reactions, including oxidation, reduction, and substitution. Common reagents used in these reactions include hydrogen selenide and potassium antimony tartrate . The major products formed from these reactions depend on the specific conditions and reagents used. For example, the reaction with hydrogen selenide gas produces pure antimony triselenide .
科学的研究の応用
Antimony selenide (Sb2Se3) has a wide range of scientific research applications. It is actively explored for use in thin-film solar cells due to its appropriate band gap, high absorption coefficient, and single-phase structure at room temperature . Additionally, it is used in the development of high-performance solar cells, where it has shown a record light-to-electricity conversion efficiency of 9.2% . The compound is also being investigated for its potential in generating hydrogen using solar energy .
類似化合物との比較
Antimony selenide (Sb2Se3) can be compared with other similar compounds such as antimony (III) oxide, antimony (III) sulfide, and antimony (III) telluride . These compounds share similar properties, such as the formal oxidation state of antimony and the covalent character of their bonding. antimony triselenide is unique in its high absorption coefficient and appropriate band gap, making it particularly suitable for photovoltaic applications .
特性
InChI |
InChI=1S/2Sb.3Se | |
---|---|---|
Source | PubChem | |
URL | https://pubchem.ncbi.nlm.nih.gov | |
Description | Data deposited in or computed by PubChem | |
InChI Key |
GNZJTRGEKSBAAS-UHFFFAOYSA-N | |
Source | PubChem | |
URL | https://pubchem.ncbi.nlm.nih.gov | |
Description | Data deposited in or computed by PubChem | |
Canonical SMILES |
[Se].[Se].[Se].[Sb].[Sb] | |
Source | PubChem | |
URL | https://pubchem.ncbi.nlm.nih.gov | |
Description | Data deposited in or computed by PubChem | |
Molecular Formula |
Sb2Se3 | |
Source | PubChem | |
URL | https://pubchem.ncbi.nlm.nih.gov | |
Description | Data deposited in or computed by PubChem | |
DSSTOX Substance ID |
DTXSID30895002 | |
Record name | Antimony triselenide | |
Source | EPA DSSTox | |
URL | https://comptox.epa.gov/dashboard/DTXSID30895002 | |
Description | DSSTox provides a high quality public chemistry resource for supporting improved predictive toxicology. | |
Molecular Weight |
480.4 g/mol | |
Source | PubChem | |
URL | https://pubchem.ncbi.nlm.nih.gov | |
Description | Data deposited in or computed by PubChem | |
CAS No. |
1315-05-5 | |
Record name | Antimony triselenide | |
Source | ChemIDplus | |
URL | https://pubchem.ncbi.nlm.nih.gov/substance/?source=chemidplus&sourceid=0001315055 | |
Description | ChemIDplus is a free, web search system that provides access to the structure and nomenclature authority files used for the identification of chemical substances cited in National Library of Medicine (NLM) databases, including the TOXNET system. | |
Record name | Antimony selenide (Sb2Se3) | |
Source | EPA Chemicals under the TSCA | |
URL | https://www.epa.gov/chemicals-under-tsca | |
Description | EPA Chemicals under the Toxic Substances Control Act (TSCA) collection contains information on chemicals and their regulations under TSCA, including non-confidential content from the TSCA Chemical Substance Inventory and Chemical Data Reporting. | |
Record name | Antimony triselenide | |
Source | EPA DSSTox | |
URL | https://comptox.epa.gov/dashboard/DTXSID30895002 | |
Description | DSSTox provides a high quality public chemistry resource for supporting improved predictive toxicology. | |
Record name | Diantimony triselenide | |
Source | European Chemicals Agency (ECHA) | |
URL | https://echa.europa.eu/substance-information/-/substanceinfo/100.013.870 | |
Description | The European Chemicals Agency (ECHA) is an agency of the European Union which is the driving force among regulatory authorities in implementing the EU's groundbreaking chemicals legislation for the benefit of human health and the environment as well as for innovation and competitiveness. | |
Explanation | Use of the information, documents and data from the ECHA website is subject to the terms and conditions of this Legal Notice, and subject to other binding limitations provided for under applicable law, the information, documents and data made available on the ECHA website may be reproduced, distributed and/or used, totally or in part, for non-commercial purposes provided that ECHA is acknowledged as the source: "Source: European Chemicals Agency, http://echa.europa.eu/". Such acknowledgement must be included in each copy of the material. ECHA permits and encourages organisations and individuals to create links to the ECHA website under the following cumulative conditions: Links can only be made to webpages that provide a link to the Legal Notice page. | |
Q1: What is the molecular formula, weight, and spectroscopic data for Antimony triselenide?
A1: Antimony triselenide (Sb2Se3) has a molecular weight of 480.64 g/mol. While a full spectroscopic analysis is beyond this Q&A, key characteristics include:
- XRD: Exhibits an orthorhombic crystal structure. [, ]
- Raman Spectroscopy: Specific phonon modes identify Sb2Se3 and distinguish it from related compounds. []
- UV-Vis Spectroscopy: Shows a band gap energy in the range of 1.1-1.2 eV, ideal for solar energy absorption. [, ]
Q2: What are the stability challenges associated with Sb2Se3 thin films?
A2: Sb2Se3 thin film properties can vary significantly depending on deposition conditions like source temperature and duration. [] Initial deposits may show higher bandgap energies (up to 1.7 eV), gradually decreasing to a stable 1.1-1.2 eV range when the source is at or above the melting point of Sb2Se3 (611 °C). [] This suggests fractionation of stoichiometric Sb2Se3 during the initial evaporation stages. []
Q3: How does the presence of oxygen affect Sb2Se3?
A3: Oxygen can be incorporated into the Sb2Se3 lattice as a defect, leading to an infrared absorption line at 527 cm-1. [] Annealing in hydrogen can passivate these oxygen defects, forming hydroxyl groups. [] The impact of oxygen on device performance needs further investigation.
Q4: What catalytic applications are being explored for Sb2Se3 nanostructures?
A4: Sb2Se3 nanowires and mesorods have demonstrated promising electrochemical hydrogen storage capabilities. [] The morphology of these nanostructures significantly influences their storage capacity. []
Q5: What makes Sb2Se3 a promising material for solar cells?
A5: Sb2Se3 possesses several desirable properties for photovoltaic applications: [, , , ]
- Suitable Band Gap: Its bandgap of 1.1-1.2 eV allows for efficient absorption of sunlight. []
- High Absorption Coefficient: It can absorb a large amount of light even in thin films, reducing material usage. [, ]
- Earth-Abundant and Non-Toxic: Unlike some other photovoltaic materials, it is composed of readily available elements. [, ]
- Good Stability: It demonstrates stability under ambient conditions, crucial for long-term device operation. [, ]
Q6: What are the main challenges limiting the efficiency of Sb2Se3 solar cells?
A6: Despite its potential, Sb2Se3 solar cells face challenges in achieving high efficiency: [, ]
- Open-Circuit Voltage Deficit: A large voltage drop exists between the theoretical and actual open-circuit voltage, hindering overall efficiency. []
- Interfacial and Bulk Recombination: Defects at the interface and within the Sb2Se3 layer can trap charge carriers, leading to recombination and reduced current. []
Q7: How can the performance of Sb2Se3 solar cells be improved?
A7: Research focuses on overcoming the limitations of Sb2Se3 solar cells through various approaches: [, , , ]
- Heterojunction Annealing: Applying a post-annealing treatment to the Sb2Se3/CdS heterojunction has been shown to reduce recombination losses and improve open-circuit voltage. []
- Back Interface Engineering: Introducing a MoO3 interlayer at the back contact in flexible Sb2Se3 devices improves crystallinity, reduces barrier height, and passivates defects, resulting in higher efficiency and flexibility. []
- Suppressing Buried Interface Recombination: Controlling the film growth kinetics and optimizing band alignment through buried interface and heterojunction engineering can minimize non-radiative recombination losses, leading to improved efficiency. []
- Doping: Introducing dopants like copper into the NiOx hole-selective layer improves the photocurrent density by blocking recombination at the back interface and facilitating hole extraction. []
Q8: What are the potential applications of flexible Sb2Se3 solar cells?
A8: Flexible Sb2Se3 solar cells hold promise for applications in: []
Q9: How is Sb2Se3 being utilized in optoelectronic devices?
A9: Sb2Se3's unique optical properties are being explored for several optoelectronic applications:
- Wavelength-Tunable Photodetectors: Grating-resonance photodetector arrays incorporating Sb2Se3 as a refractive index-tunable phase-change material demonstrate wavelength selectivity and high responsivity. []
- Reconfigurable Metasurfaces: Sb2Se3 based metasurfaces exhibit tunable focusing properties by switching between amorphous and crystalline states, enabling dynamic light manipulation. [, ]
- Polarization Rotators: Integrating Sb2Se3 with lithium niobate on insulator (LNOI) platforms enables highly efficient and tunable polarization rotation, crucial for managing polarization in integrated photonic circuits. []
Q10: How are computational methods being used to study Sb2Se3?
A10: Computational chemistry plays a crucial role in understanding and predicting the properties of Sb2Se3: [, , ]
- Defect Characterization: First-principles calculations help identify and analyze deep-level defects in Sb2Se3, linking them to stoichiometry and their impact on device performance. []
- Doping Studies: Simulations explore the impact of doping on Sb2Se3's electronic structure and optical properties, guiding experimental efforts to enhance its performance. []
- Device Simulation: Software like SCAPS-1D enables researchers to model the performance of Sb2Se3 solar cells, optimizing parameters such as absorber layer thickness and defect density to enhance efficiency. []
Q11: What are the environmental considerations related to Sb2Se3?
A11: While Sb2Se3 itself is considered relatively environmentally friendly due to its elemental composition, research on its lifecycle assessment, including synthesis, manufacturing, use, and disposal, is crucial to assess and minimize any potential environmental impact. []
Q12: What tools and resources are available for Sb2Se3 research?
A12: Various resources support research on Sb2Se3: []
Q13: What are the future research directions for Sb2Se3?
A13: Sb2Se3 research is progressing rapidly with several exciting avenues for future exploration:
Q14: What are some cross-disciplinary applications of Sb2Se3?
A14: The unique properties of Sb2Se3 make it suitable for a variety of cross-disciplinary applications: []
試験管内研究製品の免責事項と情報
BenchChemで提示されるすべての記事および製品情報は、情報提供を目的としています。BenchChemで購入可能な製品は、生体外研究のために特別に設計されています。生体外研究は、ラテン語の "in glass" に由来し、生物体の外で行われる実験を指します。これらの製品は医薬品または薬として分類されておらず、FDAから任何の医療状態、病気、または疾患の予防、治療、または治癒のために承認されていません。これらの製品を人間または動物に体内に導入する形態は、法律により厳格に禁止されています。これらのガイドラインに従うことは、研究と実験において法的および倫理的な基準の遵守を確実にするために重要です。