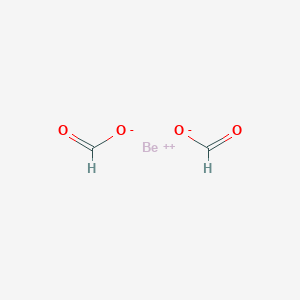
Beryllium formate
説明
Beryllium formate is an inorganic compound with the chemical formula C₂H₂BeO₄ It is a salt formed from beryllium and formic acid this compound is known for its unique properties, including its high thermal stability and its ability to form complexes with various ligands
準備方法
Synthetic Routes and Reaction Conditions: Beryllium formate can be synthesized through the reaction of beryllium hydroxide or beryllium oxide with formic acid. The reaction typically occurs under controlled conditions to ensure the complete conversion of the reactants to the desired product. The general reaction is as follows: [ \text{Be(OH)}_2 + 2 \text{HCOOH} \rightarrow \text{Be(HCOO)}_2 + 2 \text{H}_2\text{O} ]
Industrial Production Methods: Industrial production of this compound involves similar synthetic routes but on a larger scale. The process requires precise control of temperature and pH to optimize yield and purity. The use of high-purity beryllium hydroxide and formic acid is essential to minimize impurities in the final product.
Types of Reactions:
-
Oxidation: this compound can undergo oxidation reactions, particularly when exposed to strong oxidizing agents. This can lead to the formation of beryllium oxide and carbon dioxide. [ \text{Be(HCOO)}_2 + \text{O}_2 \rightarrow \text{BeO} + 2 \text{CO}_2 ]
-
Reduction: Reduction reactions involving this compound are less common but can occur under specific conditions, leading to the formation of beryllium metal and formic acid.
-
Substitution: this compound can participate in substitution reactions where the formate ions are replaced by other anions or ligands, forming new beryllium complexes.
Common Reagents and Conditions:
Oxidizing Agents: Oxygen, hydrogen peroxide, and other strong oxidizers.
Reducing Agents: Hydrogen gas, lithium aluminum hydride.
Substitution Reagents: Various ligands such as ammonia, phosphines, and other organic molecules.
Major Products Formed:
Oxidation: Beryllium oxide and carbon dioxide.
Reduction: Beryllium metal and formic acid.
Substitution: New beryllium complexes with different ligands.
科学的研究の応用
Beryllium formate has several applications in scientific research:
Chemistry: Used as a precursor for the synthesis of other beryllium compounds and complexes. It is also studied for its coordination chemistry and reactivity.
Biology: Investigated for its potential effects on biological systems, particularly in understanding beryllium toxicity and its interactions with biomolecules.
Medicine: Research into this compound’s potential use in medical imaging and as a therapeutic agent is ongoing, although its toxicity poses significant challenges.
Industry: Utilized in the production of beryllium-containing materials and as a catalyst in certain chemical reactions.
作用機序
The mechanism by which beryllium formate exerts its effects involves its ability to form stable complexes with various ligands. In biological systems, beryllium ions can interact with proteins and enzymes, potentially disrupting their function. The coordination chemistry of this compound is crucial in understanding its reactivity and interactions at the molecular level.
類似化合物との比較
Beryllium Acetate: Similar to beryllium formate but with acetate ions instead of formate. It has different solubility and reactivity properties.
Beryllium Chloride: A halide of beryllium, used in different industrial applications and has distinct chemical behavior compared to this compound.
Beryllium Nitrate: Another beryllium salt with nitrate ions, used in various chemical processes and research applications.
Uniqueness: this compound is unique due to its specific coordination chemistry and the ability to form stable complexes with a variety of ligands. Its thermal stability and reactivity with oxidizing and reducing agents also distinguish it from other beryllium compounds.
生物活性
Beryllium formate, a compound of beryllium, has garnered attention due to its unique biological activity and potential health implications. This article aims to provide a comprehensive overview of the biological effects associated with this compound, including its mechanisms of action, toxicity, and implications for human health.
This compound is characterized by its coordination with beryllium ions (Be²⁺) and formate ions (HCOO⁻). The compound can be synthesized through the reaction of beryllium hydroxide with formic acid, resulting in a white crystalline solid that is soluble in water. Understanding its chemical structure is crucial for elucidating its biological interactions.
1. Immunological Effects
Beryllium exposure has been linked to chronic beryllium disease (CBD), an immune-mediated condition that arises from sensitization to beryllium. Research indicates that this compound may exacerbate immune responses through the following mechanisms:
- Lymphocyte Activation : Beryllium can stimulate lymphocyte proliferation, as evidenced by studies using the Beryllium Lymphocyte Proliferation Test (BeLPT). In sensitized individuals, exposure to beryllium results in significant lymphocyte activation, which is critical for understanding the immunological pathways involved in CBD .
- Cytokine Production : Exposure to beryllium compounds has been shown to increase the production of pro-inflammatory cytokines. This inflammatory response is mediated by activated macrophages, which also exhibit increased phagocytic activity in the presence of beryllium .
2. Cytotoxicity and Carcinogenic Potential
Beryllium and its compounds, including this compound, have demonstrated cytotoxic effects. Key findings include:
- Cellular Transformation : Studies have shown that beryllium compounds can induce morphological changes in mammalian cells, leading to malignant transformations. This effect is particularly concerning as it suggests a potential link between beryllium exposure and cancer development .
- DNA Damage : Beryllium exposure has been associated with decreased fidelity in DNA synthesis, which may contribute to mutagenesis and tumorigenesis .
Toxicological Studies
Several studies have investigated the toxicological profile of this compound and related compounds:
Chronic Beryllium Disease (CBD)
A significant case study involved a cohort of workers exposed to beryllium in manufacturing settings. The study found that individuals with specific genetic polymorphisms (FCGR3A 158V/F) exhibited increased susceptibility to CBD. The presence of these polymorphisms correlated with greater declines in lung function over time, highlighting the interplay between genetic factors and environmental exposure .
Immunological Response Analysis
In another study focusing on immune responses, researchers observed heightened levels of CD16 expression on alveolar macrophages from patients with CBD. This increase was associated with enhanced phagocytic activity against beryllium particles, suggesting that immune system activation plays a critical role in disease progression .
特性
IUPAC Name |
beryllium;diformate | |
---|---|---|
Source | PubChem | |
URL | https://pubchem.ncbi.nlm.nih.gov | |
Description | Data deposited in or computed by PubChem | |
InChI |
InChI=1S/2CH2O2.Be/c2*2-1-3;/h2*1H,(H,2,3);/q;;+2/p-2 | |
Source | PubChem | |
URL | https://pubchem.ncbi.nlm.nih.gov | |
Description | Data deposited in or computed by PubChem | |
InChI Key |
CBKLNOZTOBKSDK-UHFFFAOYSA-L | |
Source | PubChem | |
URL | https://pubchem.ncbi.nlm.nih.gov | |
Description | Data deposited in or computed by PubChem | |
Canonical SMILES |
[Be+2].C(=O)[O-].C(=O)[O-] | |
Source | PubChem | |
URL | https://pubchem.ncbi.nlm.nih.gov | |
Description | Data deposited in or computed by PubChem | |
Molecular Formula |
Be(CHO2)2, C2H2BeO4 | |
Record name | beryllium formate | |
Source | Wikipedia | |
URL | https://en.wikipedia.org/wiki/Dictionary_of_chemical_formulas | |
Description | Chemical information link to Wikipedia. | |
Source | PubChem | |
URL | https://pubchem.ncbi.nlm.nih.gov | |
Description | Data deposited in or computed by PubChem | |
DSSTOX Substance ID |
DTXSID50149498 | |
Record name | Beryllium formate | |
Source | EPA DSSTox | |
URL | https://comptox.epa.gov/dashboard/DTXSID50149498 | |
Description | DSSTox provides a high quality public chemistry resource for supporting improved predictive toxicology. | |
Molecular Weight |
99.05 g/mol | |
Source | PubChem | |
URL | https://pubchem.ncbi.nlm.nih.gov | |
Description | Data deposited in or computed by PubChem | |
CAS No. |
1111-71-3 | |
Record name | Beryllium formate | |
Source | ChemIDplus | |
URL | https://pubchem.ncbi.nlm.nih.gov/substance/?source=chemidplus&sourceid=0001111713 | |
Description | ChemIDplus is a free, web search system that provides access to the structure and nomenclature authority files used for the identification of chemical substances cited in National Library of Medicine (NLM) databases, including the TOXNET system. | |
Record name | Beryllium formate | |
Source | EPA DSSTox | |
URL | https://comptox.epa.gov/dashboard/DTXSID50149498 | |
Description | DSSTox provides a high quality public chemistry resource for supporting improved predictive toxicology. | |
Record name | BERYLLIUM FORMATE | |
Source | FDA Global Substance Registration System (GSRS) | |
URL | https://gsrs.ncats.nih.gov/ginas/app/beta/substances/B0B3306110 | |
Description | The FDA Global Substance Registration System (GSRS) enables the efficient and accurate exchange of information on what substances are in regulated products. Instead of relying on names, which vary across regulatory domains, countries, and regions, the GSRS knowledge base makes it possible for substances to be defined by standardized, scientific descriptions. | |
Explanation | Unless otherwise noted, the contents of the FDA website (www.fda.gov), both text and graphics, are not copyrighted. They are in the public domain and may be republished, reprinted and otherwise used freely by anyone without the need to obtain permission from FDA. Credit to the U.S. Food and Drug Administration as the source is appreciated but not required. | |
試験管内研究製品の免責事項と情報
BenchChemで提示されるすべての記事および製品情報は、情報提供を目的としています。BenchChemで購入可能な製品は、生体外研究のために特別に設計されています。生体外研究は、ラテン語の "in glass" に由来し、生物体の外で行われる実験を指します。これらの製品は医薬品または薬として分類されておらず、FDAから任何の医療状態、病気、または疾患の予防、治療、または治癒のために承認されていません。これらの製品を人間または動物に体内に導入する形態は、法律により厳格に禁止されています。これらのガイドラインに従うことは、研究と実験において法的および倫理的な基準の遵守を確実にするために重要です。