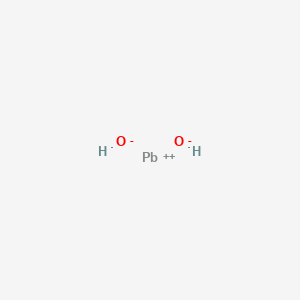
Lead(II) hydroxide
概要
説明
Lead(II) hydroxide, chemically represented as Pb(OH)₂, is an inorganic compound consisting of a lead(II) ion (Pb²⁺) and two hydroxide ions (OH⁻). It typically appears as a white amorphous powder and is known for its limited solubility in water. This compound has significant historical, scientific, and industrial relevance, despite its potential toxicity .
準備方法
Synthetic Routes and Reaction Conditions: Lead(II) hydroxide is generally produced through the reaction of lead(II) salts with an alkali. The most common method involves reacting lead(II) nitrate with sodium hydroxide, resulting in the formation of this compound and sodium nitrate: [ \text{Pb(NO}_3\text{)}_2 + 2\text{NaOH} \rightarrow \text{Pb(OH)}_2 + 2\text{NaNO}_3 ]
Industrial Production Methods: In industrial settings, this compound can be synthesized by adding a hydroxide to a solution of a lead(II) salt, such as lead(II) acetate. Careful hydrolysis of lead(II) acetate solution yields a crystalline product with the formula Pb₆O₄(OH)₄ .
化学反応の分析
Types of Reactions: Lead(II) hydroxide undergoes various chemical reactions, including:
Oxidation: Upon heating, this compound decomposes to form lead(II) oxide (PbO).
Reduction: this compound can be reduced to metallic lead under specific conditions.
Substitution: It reacts with acids to form lead(II) salts and water.
Common Reagents and Conditions:
Oxidation: Heating this compound results in the formation of lead(II) oxide. [ \text{Pb(OH)}_2 \rightarrow \text{PbO} + \text{H}_2\text{O} ]
Reduction: Reducing agents like hydrogen gas can convert this compound to metallic lead.
Substitution: Reacting with hydrochloric acid forms lead(II) chloride and water. [ \text{Pb(OH)}_2 + 2\text{HCl} \rightarrow \text{PbCl}_2 + 2\text{H}_2\text{O} ]
Major Products:
- Lead(II) oxide (PbO)
- Lead(II) chloride (PbCl₂)
- Water (H₂O)
科学的研究の応用
Lead-Acid Batteries
Lead(II) hydroxide is a crucial component in lead-acid batteries, which are widely used in automotive and industrial applications. In these batteries, Pb(OH)₂ participates in reversible reactions during charging and discharging cycles:
This reaction highlights the compound's role in energy storage and conversion processes, making it essential for the functionality of lead-acid batteries .
Wastewater Treatment
This compound is utilized in wastewater treatment due to its ability to adsorb and precipitate heavy metals and other contaminants. Its mechanism involves the formation of insoluble complexes that facilitate the removal of toxic substances from water sources .
Chemical Synthesis
In the field of chemistry, Pb(OH)₂ serves as a reagent for synthesizing other lead compounds. It can be produced through various methods, such as reacting lead(II) salts with alkaline substances:
This versatility allows it to contribute to the development of new materials and compounds .
Nanotechnology
Recent studies have explored the use of this compound nanorods in nanotechnology applications. These nanostructures exhibit unique properties that can be harnessed for advanced materials and electronic devices .
Case Study 1: Lead-Acid Battery Efficiency
Research indicates that optimizing the composition and structure of this compound within battery systems can enhance charge-discharge efficiency. Studies have shown that modifying the microstructure of Pb(OH)₂ can lead to improved performance metrics in lead-acid batteries .
Case Study 2: Heavy Metal Removal in Wastewater
A study focused on the application of this compound in treating industrial wastewater demonstrated significant reductions in heavy metal concentrations. The compound effectively precipitated contaminants, showcasing its utility in environmental remediation efforts .
作用機序
In aqueous solutions, lead(II) hydroxide acts as a weak base, forming lead(II) ions (Pb²⁺) under weakly acidic conditions. This cation hydrolyzes and, under progressively increasing alkaline conditions, forms various species, including Pb(OH)⁺, Pb(OH)₂ (aqueous), and Pb(OH)₃⁻. The compound’s ability to form these species under different pH conditions is key to its functionality in various applications .
類似化合物との比較
- Germanium(II) hydroxide (Ge(OH)₂)
- Tin(II) hydroxide (Sn(OH)₂)
- Mercury(II) hydroxide (Hg(OH)₂)
Comparison: Lead(II) hydroxide is unique due to its specific applications in lead-acid batteries and wastewater treatment. While other similar compounds like germanium(II) hydroxide, tin(II) hydroxide, and mercury(II) hydroxide share some chemical properties, they do not exhibit the same range of industrial applications. For instance, germanium(II) hydroxide and tin(II) hydroxide are more commonly used in semiconductor and electronic industries .
生物活性
Lead(II) hydroxide (Pb(OH)₂) is a compound that has garnered significant attention due to its biological activity and toxicity. This article explores the biological implications of this compound, focusing on its mechanisms of action, toxicological effects, and relevant case studies.
This compound is an inorganic compound characterized by lead in the +2 oxidation state. It can be formed through the reaction of lead(II) salts with hydroxides. In aqueous solutions, it exhibits weakly basic properties, leading to the formation of various hydroxide complexes under different pH conditions .
Mechanisms of Toxicity
Lead mimics essential metals such as calcium, zinc, and iron, disrupting numerous biological processes. Its primary mechanisms include:
- Enzymatic Inhibition : Lead inhibits delta-aminolevulinic acid dehydratase (ALAD), a crucial enzyme in heme biosynthesis. This inhibition leads to reduced heme production and subsequent anemia .
- Neurotransmitter Disruption : Lead interferes with neurotransmitter release by inhibiting calcium's binding to calmodulin and affecting NMDA receptor function, which is critical for synaptic transmission .
- Oxidative Stress : Lead exposure induces oxidative stress, leading to cellular damage through the generation of reactive oxygen species (ROS) .
Toxicological Profile
The toxicity of lead compounds, including this compound, has been extensively documented. The following table summarizes key toxicological findings related to lead exposure:
Exposure Type | Lead Levels (µg/dL) | Clinical Symptoms |
---|---|---|
Acute Poisoning | 100–120 | Muscle pain, fatigue, abdominal pain, headache, seizures |
Chronic Poisoning | 40–60 | Persistent vomiting, lethargy, convulsions |
Neurological Effects | >50 | Encephalopathy, ataxia, hyperirritability |
Gastrointestinal Effects | 60–450 | Abdominal pain, constipation, nausea |
Lead exposure can result in severe health effects across various organ systems. Notably, neurological symptoms are particularly concerning in children due to their developing brains .
Case Studies and Research Findings
Numerous studies have highlighted the biological activity and toxicity of this compound:
- Lead Exposure in Children : A study indicated that children with blood lead levels (PbB) above 80 µg/dL exhibited significant cognitive impairments and developmental delays. Acute colic was reported at levels greater than or equal to 60 µg/dL .
- Animal Studies : Research involving rats demonstrated that oral exposure to lead oxides resulted in low acute toxicity with LD50 values exceeding 2000 mg/kg body weight. However, chronic exposure led to notable neurological and gastrointestinal effects .
- Environmental Impact : A study investigated the removal of lead from wastewater using hydroxide precipitation methods. This research emphasized the importance of managing lead contamination in environmental settings due to its persistent toxicity .
特性
IUPAC Name |
lead(2+);dihydroxide | |
---|---|---|
Source | PubChem | |
URL | https://pubchem.ncbi.nlm.nih.gov | |
Description | Data deposited in or computed by PubChem | |
InChI |
InChI=1S/2H2O.Pb/h2*1H2;/q;;+2/p-2 | |
Source | PubChem | |
URL | https://pubchem.ncbi.nlm.nih.gov | |
Description | Data deposited in or computed by PubChem | |
InChI Key |
VNZYIVBHUDKWEO-UHFFFAOYSA-L | |
Source | PubChem | |
URL | https://pubchem.ncbi.nlm.nih.gov | |
Description | Data deposited in or computed by PubChem | |
Canonical SMILES |
[OH-].[OH-].[Pb+2] | |
Source | PubChem | |
URL | https://pubchem.ncbi.nlm.nih.gov | |
Description | Data deposited in or computed by PubChem | |
Molecular Formula |
H2O2Pb | |
Source | PubChem | |
URL | https://pubchem.ncbi.nlm.nih.gov | |
Description | Data deposited in or computed by PubChem | |
DSSTOX Substance ID |
DTXSID601015737 | |
Record name | Lead(2+) hydroxide | |
Source | EPA DSSTox | |
URL | https://comptox.epa.gov/dashboard/DTXSID601015737 | |
Description | DSSTox provides a high quality public chemistry resource for supporting improved predictive toxicology. | |
Molecular Weight |
241 g/mol | |
Source | PubChem | |
URL | https://pubchem.ncbi.nlm.nih.gov | |
Description | Data deposited in or computed by PubChem | |
CAS No. |
19783-14-3 | |
Record name | Lead hydroxide (Pb(OH)2) | |
Source | CAS Common Chemistry | |
URL | https://commonchemistry.cas.org/detail?cas_rn=19783-14-3 | |
Description | CAS Common Chemistry is an open community resource for accessing chemical information. Nearly 500,000 chemical substances from CAS REGISTRY cover areas of community interest, including common and frequently regulated chemicals, and those relevant to high school and undergraduate chemistry classes. This chemical information, curated by our expert scientists, is provided in alignment with our mission as a division of the American Chemical Society. | |
Explanation | The data from CAS Common Chemistry is provided under a CC-BY-NC 4.0 license, unless otherwise stated. | |
Record name | Lead(2+) hydroxide | |
Source | EPA DSSTox | |
URL | https://comptox.epa.gov/dashboard/DTXSID601015737 | |
Description | DSSTox provides a high quality public chemistry resource for supporting improved predictive toxicology. | |
Retrosynthesis Analysis
AI-Powered Synthesis Planning: Our tool employs the Template_relevance Pistachio, Template_relevance Bkms_metabolic, Template_relevance Pistachio_ringbreaker, Template_relevance Reaxys, Template_relevance Reaxys_biocatalysis model, leveraging a vast database of chemical reactions to predict feasible synthetic routes.
One-Step Synthesis Focus: Specifically designed for one-step synthesis, it provides concise and direct routes for your target compounds, streamlining the synthesis process.
Accurate Predictions: Utilizing the extensive PISTACHIO, BKMS_METABOLIC, PISTACHIO_RINGBREAKER, REAXYS, REAXYS_BIOCATALYSIS database, our tool offers high-accuracy predictions, reflecting the latest in chemical research and data.
Strategy Settings
Precursor scoring | Relevance Heuristic |
---|---|
Min. plausibility | 0.01 |
Model | Template_relevance |
Template Set | Pistachio/Bkms_metabolic/Pistachio_ringbreaker/Reaxys/Reaxys_biocatalysis |
Top-N result to add to graph | 6 |
Feasible Synthetic Routes
試験管内研究製品の免責事項と情報
BenchChemで提示されるすべての記事および製品情報は、情報提供を目的としています。BenchChemで購入可能な製品は、生体外研究のために特別に設計されています。生体外研究は、ラテン語の "in glass" に由来し、生物体の外で行われる実験を指します。これらの製品は医薬品または薬として分類されておらず、FDAから任何の医療状態、病気、または疾患の予防、治療、または治癒のために承認されていません。これらの製品を人間または動物に体内に導入する形態は、法律により厳格に禁止されています。これらのガイドラインに従うことは、研究と実験において法的および倫理的な基準の遵守を確実にするために重要です。