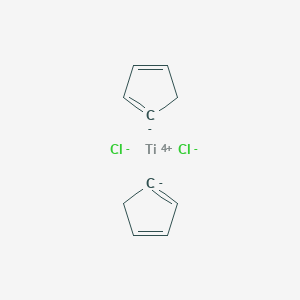
Titanocene
- 専門家チームからの見積もりを受け取るには、QUICK INQUIRYをクリックしてください。
- 品質商品を競争力のある価格で提供し、研究に集中できます。
説明
作用機序
Titanocene, also known as cyclopenta-1,3-diene;titanium(4+);dichloride, is an organotitanium compound that has been investigated for its potential use in various applications, including as an anticancer drug . This article will explore the mechanism of action of this compound, focusing on its targets, mode of action, biochemical pathways, pharmacokinetics, results of action, and the influence of environmental factors on its action.
Target of Action
This compound primarily targets cancer cells, specifically renal-cell cancer and other solid tumors . It is transported via serum albumin selectively into cancer cells and targets their DNA by coordinating strongly to phosphate groups .
Mode of Action
This compound interacts with its targets by binding to the DNA of cancer cells . This binding occurs at the phosphate groups of the DNA, leading to changes in the DNA structure . Additionally, this compound is able to induce apoptosis via the FAS receptor pathway .
Biochemical Pathways
This compound affects various biochemical pathways related to cancer cell growth and survival. Its cytotoxic and apoptosis-inducing properties lead to the death of cancer cells . Furthermore, it has anti-angiogenic properties, which means it can inhibit the formation of new blood vessels, thus preventing the supply of nutrients to the cancer cells .
Pharmacokinetics
The pharmacokinetics of this compound involve its absorption, distribution, metabolism, and excretion (ADME). After administration, this compound is transported via serum albumin selectively into cancer cells
Result of Action
The result of this compound’s action is the inhibition of cancer cell growth and the induction of apoptosis . This leads to the death of cancer cells and a reduction in tumor size. Very encouraging is the fact that this compound is breaking platinum-resistance in human colon and human lung cancer cells , which might make it attractive as a cytotoxic component of future 2nd or 3rd line cancer treatments.
Action Environment
The action of this compound can be influenced by various environmental factors. For example, the presence of serum albumin in the body can affect the transport of this compound into cancer cells
準備方法
Synthetic Routes and Reaction Conditions: Titanocene can be synthesized through several methods. One common method involves the reaction of titanium tetrachloride with cyclopentadienyl magnesium bromide in an ether solvent. The reaction proceeds as follows:
TiCl4+2C5H5MgBr→(C5H5)2TiCl2+2MgBrCl
Another method involves the reaction of titanium tetrachloride with sodium cyclopentadienide:
TiCl4+2NaC5H5→(C5H5)2TiCl2+2NaCl
Industrial Production Methods: Industrial production of this compound typically follows similar synthetic routes but on a larger scale. The reaction conditions are optimized to ensure high yield and purity of the product. The use of inert atmosphere and anhydrous conditions is crucial to prevent hydrolysis and oxidation of the reactive intermediates.
化学反応の分析
Types of Reactions: Titanocene undergoes various types of chemical reactions, including:
Reduction: this compound can be reduced to low-valent titanium species using reducing agents such as lithium aluminum hydride or sodium amalgam.
Oxidation: It can be oxidized to higher oxidation states using oxidizing agents like chlorine or bromine.
Substitution: this compound can undergo substitution reactions where the chloride ligands are replaced by other ligands such as alkyl, aryl, or alkoxide groups.
Common Reagents and Conditions:
Reduction: Lithium aluminum hydride in tetrahydrofuran.
Oxidation: Chlorine gas in dichloromethane.
Substitution: Alkyl lithium reagents in hexane.
Major Products Formed:
Reduction: Low-valent titanium complexes.
Oxidation: Higher oxidation state titanium compounds.
Substitution: this compound derivatives with various functional groups.
科学的研究の応用
Titanocene has a wide range of applications in scientific research:
Chemistry: It is used as a catalyst in various organic transformations, including polymerization and hydrogenation reactions.
Medicine: Research is ongoing to develop this compound-based drugs for cancer treatment.
類似化合物との比較
Titanocene is unique among metallocenes due to its specific reactivity and applications. Similar compounds include:
Ferrocene: Bis(cyclopentadienyl)iron(II), known for its stability and use in organic synthesis.
Zirconocene: Bis(cyclopentadienyl)zirconium(IV) dichloride, used in polymerization reactions.
Molybdenocene: Bis(cyclopentadienyl)molybdenum(IV) dichloride, known for its catalytic properties in organic transformations.
This compound stands out due to its versatility in both chemical reactions and applications, particularly in the field of medicinal chemistry.
特性
CAS番号 |
1271-19-8 |
---|---|
分子式 |
C10H2Cl2Ti-10 |
分子量 |
240.89 g/mol |
IUPAC名 |
cyclopenta-1,3-diene;dichlorotitanium |
InChI |
InChI=1S/2C5H.2ClH.Ti/c2*1-2-4-5-3-1;;;/h2*1H;2*1H;/q2*-5;;;+2/p-2 |
InChIキー |
DYUFLUNZOBVZGN-UHFFFAOYSA-L |
SMILES |
C1C=CC=[C-]1.C1C=CC=[C-]1.[Cl-].[Cl-].[Ti+4] |
正規SMILES |
[CH-]1[C-]=[C-][C-]=[C-]1.[CH-]1[C-]=[C-][C-]=[C-]1.Cl[Ti]Cl |
Color/Form |
Bright red acicular crystals from toluene Reddish-orange crystalline solid |
密度 |
1.6 (NTP, 1992) 1.60 |
melting_point |
552 °F (NTP, 1992) 289 °C +/- 2 deg |
1271-19-8 | |
物理的記述 |
Titanocene dichloride appears as red to red-orange crystals. (NTP, 1992) |
ピクトグラム |
Acute Toxic; Irritant; Health Hazard |
賞味期限 |
STABLE IN DRY AIR; SLOWLY HYDROLYZED IN MOIST AIR |
溶解性 |
Decomposes (NTP, 1992) Moderately sol in toluene, chloroform, alc, other hydroxylic solvents; sparingly sol in water, petroleum ether, benzene, ether, carbon disulfide, carbon tetrachloride |
製品の起源 |
United States |
試験管内研究製品の免責事項と情報
BenchChemで提示されるすべての記事および製品情報は、情報提供を目的としています。BenchChemで購入可能な製品は、生体外研究のために特別に設計されています。生体外研究は、ラテン語の "in glass" に由来し、生物体の外で行われる実験を指します。これらの製品は医薬品または薬として分類されておらず、FDAから任何の医療状態、病気、または疾患の予防、治療、または治癒のために承認されていません。これらの製品を人間または動物に体内に導入する形態は、法律により厳格に禁止されています。これらのガイドラインに従うことは、研究と実験において法的および倫理的な基準の遵守を確実にするために重要です。