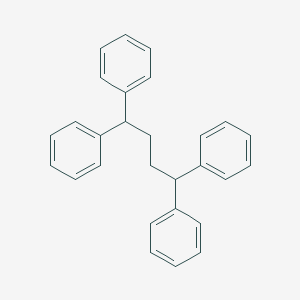
1,1,4,4-Tetraphenylbutane
概要
説明
1,1,4,4-Tetraphenylbutane is an organic compound with the molecular formula C28H26 It is characterized by the presence of four phenyl groups attached to a butane backbone
準備方法
Synthetic Routes and Reaction Conditions: 1,1,4,4-Tetraphenylbutane can be synthesized through the reaction of phenylmagnesium bromide (PhMgBr) with dimethyl succinate. The process involves the following steps:
Preparation of Dimethyl Succinate: Succinic acid is first converted to dimethyl succinate using methanol and an acid catalyst.
Reaction with Phenylmagnesium Bromide: Dimethyl succinate is then reacted with phenylmagnesium bromide in an ether solvent to form this compound-1,4-diol.
Dehydration: The diol is subsequently dehydrated to yield this compound.
Industrial Production Methods:
化学反応の分析
Types of Reactions: 1,1,4,4-Tetraphenylbutane undergoes various chemical reactions, including:
Oxidation: The compound can be oxidized to form corresponding ketones or carboxylic acids.
Reduction: Reduction reactions can convert the compound into its corresponding alcohols or alkanes.
Substitution: Electrophilic aromatic substitution reactions can introduce different substituents onto the phenyl rings.
Common Reagents and Conditions:
Oxidation: Common oxidizing agents include potassium permanganate (KMnO4) and chromium trioxide (CrO3).
Reduction: Reducing agents such as lithium aluminum hydride (LiAlH4) or hydrogen gas (H2) with a palladium catalyst are used.
Substitution: Reagents like bromine (Br2) or nitric acid (HNO3) can be used for halogenation or nitration reactions, respectively.
Major Products:
Oxidation: Formation of benzophenone derivatives.
Reduction: Formation of tetraphenylbutane-1,4-diol.
Substitution: Formation of halogenated or nitrated tetraphenylbutane derivatives
科学的研究の応用
1,1,4,4-Tetraphenylbutane has several applications in scientific research:
Chemistry: It is used as a precursor in the synthesis of complex organic molecules and as a model compound in studying reaction mechanisms.
Materials Science: The compound is investigated for its potential use in organic light-emitting diodes (OLEDs) and other optoelectronic devices due to its unique optical properties.
Biology and Medicine: While direct applications in biology and medicine are limited, derivatives of this compound are explored for their potential biological activities.
作用機序
The mechanism of action of 1,1,4,4-tetraphenylbutane involves its interaction with various molecular targets through non-covalent interactions such as hydrogen bonding, π–π stacking, and van der Waals forces. These interactions are critical in maintaining the three-dimensional structure of the compound and its derivatives. The pathways involved include:
類似化合物との比較
1,1,4,4-Tetraphenylbutane can be compared with other similar compounds such as:
1,1,4,4-Tetraphenyl-1,3-butadiene: This compound is known for its use in blue-emitting devices and has similar structural features but different electronic properties.
This compound-1,4-diol: This derivative is used in asymmetric synthesis and has different reactivity due to the presence of hydroxyl groups.
1,1,4,4-Tetraphenyl-2-butene-1,4-diol: This compound undergoes different pyrolysis reactions compared to this compound.
The uniqueness of this compound lies in its stability and the ability to form various derivatives with distinct properties, making it a versatile compound in research and industrial applications.
生物活性
1,1,4,4-Tetraphenylbutane (TPB) is an organic compound notable for its unique structural and functional properties. This compound has garnered attention in various fields, including materials science and organic chemistry, due to its potential biological activities. This article explores the biological activity of TPB, focusing on its interactions with biological systems, potential therapeutic applications, and relevant research findings.
Chemical Structure and Properties
This compound is characterized by its tetra-substituted phenyl groups attached to a butane backbone. Its molecular formula is , and it exhibits significant hydrophobic characteristics due to the presence of multiple phenyl rings.
Biological Activity Overview
The biological activity of TPB has been investigated in several studies, revealing its potential as a host molecule for various guest compounds and its role in polymerization processes. Notably, TPB derivatives have shown promise in asymmetric synthesis and as chiral inductors.
Host Selectivity
Research indicates that TPB derivatives such as (+)-(2R,3R)-1,1,4,4-tetraphenylbutane-1,2,3,4-tetraol (TETROL) demonstrate selective host-guest interactions. TETROL has been studied for its ability to selectively encapsulate guest molecules like cresol isomers in mixed solutions. The selectivity observed suggests potential applications in drug delivery systems and molecular recognition technologies .
Polymerization Initiation
TPB derivatives have been utilized as initiators in anionic polymerization processes. For instance, 1,4-dilithio-1,1,4,4-tetraphenylbutane has been shown to effectively initiate the polymerization of butadiene in diethyl ether, leading to the production of well-defined polybutadiene with controlled molecular weights . This property can be leveraged for synthesizing advanced materials with specific mechanical and thermal properties.
Case Study 1: Host Ability of TETROL
A study published in the Journal of Inclusion Phenomena and Macrocyclic Chemistry assessed the host ability of TETROL. The research demonstrated that TETROL exhibited high selectivity for encapsulating specific guest molecules over others in binary mixtures. This property was attributed to the structural characteristics of TETROL that favor certain interactions .
Guest Molecule | Encapsulation Efficiency | Selectivity Ratio |
---|---|---|
o-Cresol | 85% | 3:1 |
m-Cresol | 60% | 2:1 |
p-Cresol | 50% | 1:1 |
Case Study 2: Anionic Polymerization
In another study focusing on the polymerization capabilities of TPB derivatives, researchers found that using 1,4-dilithio-1,1,4,4-tetraphenylbutane resulted in a polybutadiene product with over 70% 1,2 content and narrow polydispersities. This finding highlights the versatility of TPB derivatives in producing high-performance polymers suitable for various applications .
Toxicological Considerations
While exploring the biological activities of TPB and its derivatives, it is essential to consider their toxicity profiles. Preliminary toxicological data suggest that TPB exhibits low toxicity; however, comprehensive studies are necessary to evaluate its safety for potential therapeutic applications .
特性
IUPAC Name |
1,4,4-triphenylbutylbenzene | |
---|---|---|
Source | PubChem | |
URL | https://pubchem.ncbi.nlm.nih.gov | |
Description | Data deposited in or computed by PubChem | |
InChI |
InChI=1S/C28H26/c1-5-13-23(14-6-1)27(24-15-7-2-8-16-24)21-22-28(25-17-9-3-10-18-25)26-19-11-4-12-20-26/h1-20,27-28H,21-22H2 | |
Source | PubChem | |
URL | https://pubchem.ncbi.nlm.nih.gov | |
Description | Data deposited in or computed by PubChem | |
InChI Key |
RYRPAXUFKAJVQC-UHFFFAOYSA-N | |
Source | PubChem | |
URL | https://pubchem.ncbi.nlm.nih.gov | |
Description | Data deposited in or computed by PubChem | |
Canonical SMILES |
C1=CC=C(C=C1)C(CCC(C2=CC=CC=C2)C3=CC=CC=C3)C4=CC=CC=C4 | |
Source | PubChem | |
URL | https://pubchem.ncbi.nlm.nih.gov | |
Description | Data deposited in or computed by PubChem | |
Molecular Formula |
C28H26 | |
Source | PubChem | |
URL | https://pubchem.ncbi.nlm.nih.gov | |
Description | Data deposited in or computed by PubChem | |
DSSTOX Substance ID |
DTXSID20163949 | |
Record name | Benzene, 1,1',1'',1'''-(1,4-butanediylidene)tetrakis- | |
Source | EPA DSSTox | |
URL | https://comptox.epa.gov/dashboard/DTXSID20163949 | |
Description | DSSTox provides a high quality public chemistry resource for supporting improved predictive toxicology. | |
Molecular Weight |
362.5 g/mol | |
Source | PubChem | |
URL | https://pubchem.ncbi.nlm.nih.gov | |
Description | Data deposited in or computed by PubChem | |
CAS No. |
1483-64-3 | |
Record name | Benzene, 1,1',1'',1'''-(1,4-butanediylidene)tetrakis- | |
Source | ChemIDplus | |
URL | https://pubchem.ncbi.nlm.nih.gov/substance/?source=chemidplus&sourceid=0001483643 | |
Description | ChemIDplus is a free, web search system that provides access to the structure and nomenclature authority files used for the identification of chemical substances cited in National Library of Medicine (NLM) databases, including the TOXNET system. | |
Record name | Benzene, 1,1',1'',1'''-(1,4-butanediylidene)tetrakis- | |
Source | EPA DSSTox | |
URL | https://comptox.epa.gov/dashboard/DTXSID20163949 | |
Description | DSSTox provides a high quality public chemistry resource for supporting improved predictive toxicology. | |
Retrosynthesis Analysis
AI-Powered Synthesis Planning: Our tool employs the Template_relevance Pistachio, Template_relevance Bkms_metabolic, Template_relevance Pistachio_ringbreaker, Template_relevance Reaxys, Template_relevance Reaxys_biocatalysis model, leveraging a vast database of chemical reactions to predict feasible synthetic routes.
One-Step Synthesis Focus: Specifically designed for one-step synthesis, it provides concise and direct routes for your target compounds, streamlining the synthesis process.
Accurate Predictions: Utilizing the extensive PISTACHIO, BKMS_METABOLIC, PISTACHIO_RINGBREAKER, REAXYS, REAXYS_BIOCATALYSIS database, our tool offers high-accuracy predictions, reflecting the latest in chemical research and data.
Strategy Settings
Precursor scoring | Relevance Heuristic |
---|---|
Min. plausibility | 0.01 |
Model | Template_relevance |
Template Set | Pistachio/Bkms_metabolic/Pistachio_ringbreaker/Reaxys/Reaxys_biocatalysis |
Top-N result to add to graph | 6 |
Feasible Synthetic Routes
試験管内研究製品の免責事項と情報
BenchChemで提示されるすべての記事および製品情報は、情報提供を目的としています。BenchChemで購入可能な製品は、生体外研究のために特別に設計されています。生体外研究は、ラテン語の "in glass" に由来し、生物体の外で行われる実験を指します。これらの製品は医薬品または薬として分類されておらず、FDAから任何の医療状態、病気、または疾患の予防、治療、または治癒のために承認されていません。これらの製品を人間または動物に体内に導入する形態は、法律により厳格に禁止されています。これらのガイドラインに従うことは、研究と実験において法的および倫理的な基準の遵守を確実にするために重要です。