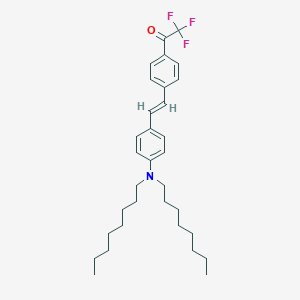
Chromoionophore X
説明
Chromoionophore X, also known as 4-Dioctylamino-4′-(trifluoroacetyl)stilbene or ETH 4004, is a chemical compound with the empirical formula C32H44F3NO . It is used in the detection of alcohol .
Molecular Structure Analysis
The molecular weight of this compound is 515.69 . Its structure includes a hydrophilic center and a hydrophobic portion that interacts with the membrane .Chemical Reactions Analysis
This compound, like other ionophores, is involved in complexation reactions that do not directly result in an optical signal change . Signal transducers are thus required for carrier-based ion-selective optical sensors .Physical And Chemical Properties Analysis
This compound is a highly lipophilic pH indicator . It’s known to be pH dependent and usually operated in a passive mode .科学的研究の応用
Signal Control by Metal Ionic Inputs : Chromoionophore-based calix[4]crown demonstrates an 'off-on-off' signaling for metal cationic inputs, useful for molecular information processing and designing new sensory materials (Kubo, Obara, & Tokita, 1999).
Spectropotentiometry in Ion-Selective Membranes : A spectropotentiometric imaging technique using chromoionophores helps in studying transport processes and cation interference in H+-selective liquid polymer membranes (Schneider et al., 1996).
Development for Integrated Optical Sensors : New chromoionophores focused on Near-Infrared (NIR) applications have been developed for use in monolithically integrated optical sensors (Citterio, Rásonyi, & Spichiger, 1996).
Monomolecular Layer Formation : Chromoionophore I forms Langmuir-Blodgett films, with stability depending on subphase pH and the degree of protonation, indicating its potential as a template for densely packed protein arrays (Ishii, 2009).
Optical Sensor for Uranyl Ion Detection : Mono(indoaniline)-derived calix[6]arene chromoionophores show selective UO22+ ion-induced color changes, suggesting their potential as optical sensors for UO22+ detection (Kubo, Maeda, Nakamura, & Tokita, 1994).
Potassium Selective Chromoionophores : Chromoionophores synthesised from simple precursors show potential for use in optical fibre sensors for potassium, especially in the presence of other ions (Danks & Sutherland, 1992).
Crown Ethers and Related Structures for Ion Sensing : The design concept and photometric function of chromoionophores based on spherand, hemispherand, cryptand, dibenzo-16-crown-5, and calix[4]crown for alkali metal ion sensing in aqueous media are highlighted (Hayashita et al., 1998).
作用機序
将来の方向性
Recent advances aim to renovate the chromoionophores and detection modes to overcome the pH cross-response and to eliminate the background optical interference . Topics include sensors based on solvatochromic dyes, alternative chromoionophores, photoswitchable sensors, upconverting nanoparticles, luminescence decay time, and others .
特性
IUPAC Name |
1-[4-[(E)-2-[4-(dioctylamino)phenyl]ethenyl]phenyl]-2,2,2-trifluoroethanone | |
---|---|---|
Source | PubChem | |
URL | https://pubchem.ncbi.nlm.nih.gov | |
Description | Data deposited in or computed by PubChem | |
InChI |
InChI=1S/C32H44F3NO/c1-3-5-7-9-11-13-25-36(26-14-12-10-8-6-4-2)30-23-19-28(20-24-30)16-15-27-17-21-29(22-18-27)31(37)32(33,34)35/h15-24H,3-14,25-26H2,1-2H3/b16-15+ | |
Source | PubChem | |
URL | https://pubchem.ncbi.nlm.nih.gov | |
Description | Data deposited in or computed by PubChem | |
InChI Key |
LQCOAQAROPQVJL-FOCLMDBBSA-N | |
Source | PubChem | |
URL | https://pubchem.ncbi.nlm.nih.gov | |
Description | Data deposited in or computed by PubChem | |
Canonical SMILES |
CCCCCCCCN(CCCCCCCC)C1=CC=C(C=C1)C=CC2=CC=C(C=C2)C(=O)C(F)(F)F | |
Source | PubChem | |
URL | https://pubchem.ncbi.nlm.nih.gov | |
Description | Data deposited in or computed by PubChem | |
Isomeric SMILES |
CCCCCCCCN(CCCCCCCC)C1=CC=C(C=C1)/C=C/C2=CC=C(C=C2)C(=O)C(F)(F)F | |
Source | PubChem | |
URL | https://pubchem.ncbi.nlm.nih.gov | |
Description | Data deposited in or computed by PubChem | |
Molecular Formula |
C32H44F3NO | |
Source | PubChem | |
URL | https://pubchem.ncbi.nlm.nih.gov | |
Description | Data deposited in or computed by PubChem | |
DSSTOX Substance ID |
DTXSID30421744 | |
Record name | Chromoionophore X | |
Source | EPA DSSTox | |
URL | https://comptox.epa.gov/dashboard/DTXSID30421744 | |
Description | DSSTox provides a high quality public chemistry resource for supporting improved predictive toxicology. | |
Molecular Weight |
515.7 g/mol | |
Source | PubChem | |
URL | https://pubchem.ncbi.nlm.nih.gov | |
Description | Data deposited in or computed by PubChem | |
CAS No. |
192190-92-4 | |
Record name | Chromoionophore X | |
Source | EPA DSSTox | |
URL | https://comptox.epa.gov/dashboard/DTXSID30421744 | |
Description | DSSTox provides a high quality public chemistry resource for supporting improved predictive toxicology. | |
Retrosynthesis Analysis
AI-Powered Synthesis Planning: Our tool employs the Template_relevance Pistachio, Template_relevance Bkms_metabolic, Template_relevance Pistachio_ringbreaker, Template_relevance Reaxys, Template_relevance Reaxys_biocatalysis model, leveraging a vast database of chemical reactions to predict feasible synthetic routes.
One-Step Synthesis Focus: Specifically designed for one-step synthesis, it provides concise and direct routes for your target compounds, streamlining the synthesis process.
Accurate Predictions: Utilizing the extensive PISTACHIO, BKMS_METABOLIC, PISTACHIO_RINGBREAKER, REAXYS, REAXYS_BIOCATALYSIS database, our tool offers high-accuracy predictions, reflecting the latest in chemical research and data.
Strategy Settings
Precursor scoring | Relevance Heuristic |
---|---|
Min. plausibility | 0.01 |
Model | Template_relevance |
Template Set | Pistachio/Bkms_metabolic/Pistachio_ringbreaker/Reaxys/Reaxys_biocatalysis |
Top-N result to add to graph | 6 |
Feasible Synthetic Routes
試験管内研究製品の免責事項と情報
BenchChemで提示されるすべての記事および製品情報は、情報提供を目的としています。BenchChemで購入可能な製品は、生体外研究のために特別に設計されています。生体外研究は、ラテン語の "in glass" に由来し、生物体の外で行われる実験を指します。これらの製品は医薬品または薬として分類されておらず、FDAから任何の医療状態、病気、または疾患の予防、治療、または治癒のために承認されていません。これらの製品を人間または動物に体内に導入する形態は、法律により厳格に禁止されています。これらのガイドラインに従うことは、研究と実験において法的および倫理的な基準の遵守を確実にするために重要です。