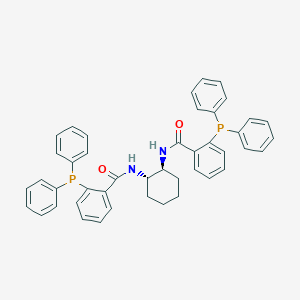
1S,2S-Dhac-phenyl trost ligand
- 専門家チームからの見積もりを受け取るには、QUICK INQUIRYをクリックしてください。
- 品質商品を競争力のある価格で提供し、研究に集中できます。
説明
準備方法
The synthesis of 1S,2S-Dhac-phenyl Trost ligand involves several steps:
Starting Materials: The synthesis begins with (1S,2S)-1,2-diaminocyclohexane and 2-diphenylphosphinobenzoyl chloride.
Reaction Conditions: The reaction typically occurs in the presence of a base such as triethylamine, which facilitates the formation of the desired ligand by neutralizing the hydrochloric acid byproduct.
Industrial Production: On an industrial scale, the production of this ligand involves similar steps but with optimized conditions to ensure high yield and purity.
化学反応の分析
1S,2S-Dhac-phenyl Trost ligand is known for its role in asymmetric allylic alkylation reactions. These reactions are crucial for forming carbon-carbon bonds in a stereoselective manner. The ligand coordinates with transition metals such as palladium, forming a complex that facilitates the alkylation process.
Types of Reactions: The ligand primarily undergoes coordination with transition metals, enabling it to participate in catalytic cycles.
Common Reagents and Conditions: Typical reagents include allylic substrates, nucleophiles, and palladium catalysts. The reactions are often carried out under inert atmospheres to prevent oxidation.
科学的研究の応用
1S,2S-Dhac-phenyl Trost ligand has a wide range of applications in scientific research:
Chemistry: It is extensively used in asymmetric synthesis to produce enantiomerically pure compounds.
Biology: The ligand’s ability to facilitate the synthesis of chiral molecules makes it valuable in the development of biologically active compounds.
Medicine: It plays a crucial role in the synthesis of chiral drugs, which often exhibit improved efficacy and reduced side effects compared to their racemic counterparts.
作用機序
The mechanism by which 1S,2S-Dhac-phenyl Trost ligand exerts its effects involves the formation of a chiral environment around the metal center. This chiral environment induces enantioselectivity in the catalytic reactions. The ligand coordinates with the metal through its phosphine groups, while the cyclohexane backbone provides the necessary steric hindrance to control the orientation of the substrates .
類似化合物との比較
1S,2S-Dhac-phenyl Trost ligand is unique due to its high enantioselectivity and versatility in various catalytic reactions. Similar compounds include:
(R,R)-DACH-phenyl Trost ligand: This ligand has the opposite chirality and is used in similar asymmetric reactions.
(S,S)-DACH-naphthyl Trost ligand: This ligand has a naphthyl group instead of a phenyl group, offering different steric and electronic properties.
(R,R)-DACH-naphthyl Trost ligand: Similar to the (S,S)-DACH-naphthyl Trost ligand but with opposite chirality.
These ligands share similar structural features but differ in their chiral centers and substituents, which can significantly impact their catalytic performance and selectivity.
特性
IUPAC Name |
2-diphenylphosphanyl-N-[(1S,2S)-2-[(2-diphenylphosphanylbenzoyl)amino]cyclohexyl]benzamide |
Source
|
---|---|---|
Source | PubChem | |
URL | https://pubchem.ncbi.nlm.nih.gov | |
Description | Data deposited in or computed by PubChem | |
InChI |
InChI=1S/C44H40N2O2P2/c47-43(37-27-13-17-31-41(37)49(33-19-5-1-6-20-33)34-21-7-2-8-22-34)45-39-29-15-16-30-40(39)46-44(48)38-28-14-18-32-42(38)50(35-23-9-3-10-24-35)36-25-11-4-12-26-36/h1-14,17-28,31-32,39-40H,15-16,29-30H2,(H,45,47)(H,46,48)/t39-,40-/m0/s1 |
Source
|
Source | PubChem | |
URL | https://pubchem.ncbi.nlm.nih.gov | |
Description | Data deposited in or computed by PubChem | |
InChI Key |
AXMSEDAJMGFTLR-ZAQUEYBZSA-N |
Source
|
Source | PubChem | |
URL | https://pubchem.ncbi.nlm.nih.gov | |
Description | Data deposited in or computed by PubChem | |
Canonical SMILES |
C1CCC(C(C1)NC(=O)C2=CC=CC=C2P(C3=CC=CC=C3)C4=CC=CC=C4)NC(=O)C5=CC=CC=C5P(C6=CC=CC=C6)C7=CC=CC=C7 |
Source
|
Source | PubChem | |
URL | https://pubchem.ncbi.nlm.nih.gov | |
Description | Data deposited in or computed by PubChem | |
Isomeric SMILES |
C1CC[C@@H]([C@H](C1)NC(=O)C2=CC=CC=C2P(C3=CC=CC=C3)C4=CC=CC=C4)NC(=O)C5=CC=CC=C5P(C6=CC=CC=C6)C7=CC=CC=C7 |
Source
|
Source | PubChem | |
URL | https://pubchem.ncbi.nlm.nih.gov | |
Description | Data deposited in or computed by PubChem | |
Molecular Formula |
C44H40N2O2P2 |
Source
|
Source | PubChem | |
URL | https://pubchem.ncbi.nlm.nih.gov | |
Description | Data deposited in or computed by PubChem | |
Molecular Weight |
690.7 g/mol |
Source
|
Source | PubChem | |
URL | https://pubchem.ncbi.nlm.nih.gov | |
Description | Data deposited in or computed by PubChem | |
試験管内研究製品の免責事項と情報
BenchChemで提示されるすべての記事および製品情報は、情報提供を目的としています。BenchChemで購入可能な製品は、生体外研究のために特別に設計されています。生体外研究は、ラテン語の "in glass" に由来し、生物体の外で行われる実験を指します。これらの製品は医薬品または薬として分類されておらず、FDAから任何の医療状態、病気、または疾患の予防、治療、または治癒のために承認されていません。これらの製品を人間または動物に体内に導入する形態は、法律により厳格に禁止されています。これらのガイドラインに従うことは、研究と実験において法的および倫理的な基準の遵守を確実にするために重要です。