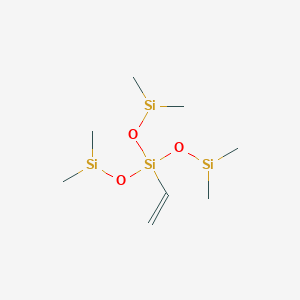
Vinyl tris(dimethylsiloxy)silane
- 専門家チームからの見積もりを受け取るには、QUICK INQUIRYをクリックしてください。
- 品質商品を競争力のある価格で提供し、研究に集中できます。
説明
Vinyl tris(dimethylsiloxy)silane, also known as 3-[(dimethylsilyl)oxy]-3-ethenyl-1,1,5,5-tetramethyltrisiloxane, is an organosilicon compound with the molecular formula C8H24O3Si4. This compound is characterized by its vinyl group attached to a silicon atom, which is further bonded to three dimethylsiloxy groups. It is a colorless liquid with a boiling point of 204°C and a density of 0.79 g/cm³ [2][2].
準備方法
Vinyl tris(dimethylsiloxy)silane can be synthesized through various methods. One common synthetic route involves the reaction of vinyltrichlorosilane with hexamethyldisiloxane in the presence of a catalyst. The reaction typically proceeds under mild conditions, yielding the desired product after purification . Industrial production methods often involve similar processes but are optimized for higher yields and purity. For instance, the use of methanol and water as solvents, along with specific catalysts, can enhance the efficiency of the reaction .
化学反応の分析
Vinyl tris(dimethylsiloxy)silane undergoes several types of chemical reactions, including:
科学的研究の応用
Introduction to Vinyl Tris(dimethylsiloxy)silane
This compound is a versatile organosilicon compound that has garnered attention in various fields, particularly in materials science, organic synthesis, and biomedical applications. Its unique chemical structure, characterized by vinyl and siloxy groups, allows for significant reactivity and functionalization, making it an essential component in the development of advanced materials.
Materials Science
Silicone Elastomers : this compound is used as a precursor for silicone elastomers. The incorporation of vinyl groups allows for cross-linking reactions that enhance the mechanical properties of silicone rubbers. This application is critical in industries requiring durable and flexible materials, such as automotive and consumer goods.
Coatings and Sealants : The compound is also utilized in formulating advanced coatings and sealants that exhibit excellent adhesion, water repellency, and thermal stability. These characteristics are essential for applications in construction and automotive sectors.
Organic Synthesis
Hiyama Coupling Reactions : this compound serves as a substrate in Hiyama coupling reactions, providing a stereochemical alternative to traditional methods. This application is particularly useful for synthesizing complex organic molecules with specific stereochemistry .
Functionalization of Surfaces : The compound can be used to modify surfaces through silanization processes, enhancing properties such as hydrophobicity and biocompatibility. This is especially relevant in the development of biomedical devices and sensors.
Biomedical Applications
Drug Delivery Systems : Research indicates that this compound can be incorporated into drug delivery systems due to its ability to form stable siloxane networks. These networks can encapsulate therapeutic agents, improving their stability and release profiles.
Tissue Engineering : The compound's biocompatibility makes it suitable for use in tissue engineering scaffolds. Its ability to support cell adhesion and proliferation is crucial for developing functional tissue constructs.
Case Study 1: Synthesis of Silicone Elastomers
A study demonstrated the synthesis of hydride-terminated vinyl-containing silicone elastomers using this compound as a key monomer. The resulting elastomers exhibited enhanced mechanical properties due to effective cross-linking facilitated by the vinyl groups .
Case Study 2: Functionalization of Biomedical Devices
In another investigation, researchers utilized this compound to modify the surfaces of medical implants. The treatment improved the hydrophobicity and biocompatibility of the implants, leading to better integration with biological tissues .
作用機序
The mechanism of action of vinyl tris(dimethylsiloxy)silane primarily involves its ability to undergo hydrosilylation and other addition reactions. The vinyl group acts as a reactive site for electrophilic addition, while the dimethylsiloxy groups provide steric protection and stability to the molecule. This combination allows the compound to participate in various chemical transformations, leading to the formation of complex organosilicon structures .
類似化合物との比較
Vinyl tris(dimethylsiloxy)silane can be compared with other similar compounds such as tris(trimethylsiloxy)silane and tris(dimethylvinylsiloxy)silane. While all these compounds contain silicon-oxygen bonds and vinyl groups, their reactivity and applications differ:
Tris(trimethylsiloxy)silane: This compound has three trimethylsiloxy groups instead of dimethylsiloxy groups.
Tris(dimethylvinylsiloxy)silane: This compound has vinyl groups attached to each silicon atom, increasing its reactivity in polymerization reactions. It is often used in the synthesis of highly branched poly(siloxysilane) polymers.
生物活性
Vinyl tris(dimethylsiloxy)silane is a siloxane compound that has garnered attention for its potential applications in various fields, including materials science and biomedicine. This article explores the biological activity of this compound, focusing on its safety, toxicity, and potential biological interactions based on diverse research findings.
This compound is characterized by its siloxane backbone, which contributes to its unique properties. The molecular formula is C6H18O3Si4, and it has a molecular weight of approximately 330.68 g/mol. Its structure features three dimethylsiloxy groups attached to a vinyl group, which enhances its reactivity in polymerization processes and cross-linking applications .
Toxicity and Safety
Research indicates that siloxanes, including this compound, generally exhibit low toxicity. A comprehensive study on siloxanes highlighted that these compounds are not irritating to skin or eyes and do not show significant sensitization effects upon skin contact . However, specific studies have raised concerns regarding potential endocrine disruption and reproductive toxicity associated with some siloxanes, particularly D4 and D5 variants. These concerns stem from their persistence in the environment and their ability to bioaccumulate .
Genotoxicity
Current literature suggests that this compound does not exhibit genotoxic effects in vitro or in vivo. Studies have shown that various siloxanes do not induce mutations or chromosomal damage in standard assays, which is crucial for assessing their safety for biological applications .
1. Application in Medical Coatings
A notable study investigated the use of this compound in non-self-adhesive coatings for medical devices. These coatings are designed to protect biological surfaces from mechanical stress while maintaining biocompatibility. The findings indicated that the coatings effectively reduced friction and wear on surfaces exposed to biological fluids, suggesting a promising application for this siloxane compound in medical technology .
2. Biocompatibility Assessments
In another case study focusing on biocompatibility, researchers evaluated the interaction of this compound with human skin fibroblasts. The results demonstrated that the compound did not adversely affect cell viability or proliferation, indicating its potential as a safe material for biomedical applications .
Summary of Research Findings
The following table summarizes key findings related to the biological activity of this compound:
特性
InChI |
InChI=1S/C8H21O3Si4/c1-8-15(9-12(2)3,10-13(4)5)11-14(6)7/h8H,1H2,2-7H3 |
Source
|
---|---|---|
Source | PubChem | |
URL | https://pubchem.ncbi.nlm.nih.gov | |
Description | Data deposited in or computed by PubChem | |
InChI Key |
QMYWTTZYMZXKNP-UHFFFAOYSA-N |
Source
|
Source | PubChem | |
URL | https://pubchem.ncbi.nlm.nih.gov | |
Description | Data deposited in or computed by PubChem | |
Canonical SMILES |
C[Si](C)O[Si](C=C)(O[Si](C)C)O[Si](C)C |
Source
|
Source | PubChem | |
URL | https://pubchem.ncbi.nlm.nih.gov | |
Description | Data deposited in or computed by PubChem | |
Molecular Formula |
C8H21O3Si4 |
Source
|
Source | PubChem | |
URL | https://pubchem.ncbi.nlm.nih.gov | |
Description | Data deposited in or computed by PubChem | |
Molecular Weight |
277.59 g/mol |
Source
|
Source | PubChem | |
URL | https://pubchem.ncbi.nlm.nih.gov | |
Description | Data deposited in or computed by PubChem | |
試験管内研究製品の免責事項と情報
BenchChemで提示されるすべての記事および製品情報は、情報提供を目的としています。BenchChemで購入可能な製品は、生体外研究のために特別に設計されています。生体外研究は、ラテン語の "in glass" に由来し、生物体の外で行われる実験を指します。これらの製品は医薬品または薬として分類されておらず、FDAから任何の医療状態、病気、または疾患の予防、治療、または治癒のために承認されていません。これらの製品を人間または動物に体内に導入する形態は、法律により厳格に禁止されています。これらのガイドラインに従うことは、研究と実験において法的および倫理的な基準の遵守を確実にするために重要です。